Synthesis and photocatalysis of
Nano-TiO2-SnO2 mesoporous material
Ding Shiwen, Zhang Hongjun, Gao Lan, Zhang Yuanyuan
(College of Chemistry and Environmental Science, Hebei University Baoding 071002 China)
Abstract Nanometric TiO2-SnO2
compound mesoporous material was prepared by normal pressure
liquid-phase reaction, using TiCl4 and SnCl4·5H2O as raw
material and nanometric active carbon as template, PEG-6000 as structure directing agent.
This method utilized the positional hindrance effect of the long chain of PEG-6000 in
space. XRD analysis showed that the transformation temperature of the nanocrystalline TiO2
from anatase phase to rutile phase decreases with Sn-doping. TEM
measurement indicated that the TiO2-SnO2 was spherical particles,
and the average diameter of the particles was 15~20nm. N2 adsorption-desorption
isotherms indicated that the sample was typical mesoporous material. EDS measurement
indicated that the found Ti:Sn value of the sample agreed with the original mixing ratio
and the uniformity was good. The adsorption and photocatalytic experiments showed that the
material had the best photocatalytic reactivity when the content of Sn was 4 %, the
adsorption of which increased 6.6 % relative to that of P25. After being irradiated under
the sunlight for 40 min, the compound could completely degrade the acid red 3R dye.
Keywords nano- TiO2-SnO2; normal pressure liquid-phase
reaction; mesoporous; photocatalysis
Owing to its chemical
stability, nontoxicity and chemical and photochemical anticorruption, TiO2 is
an important semiconductor of photo-catalytic material, which plays an important role in
wastewater treatment and air decontamination[1¨D3]. But pure TiO2 is a broad bandgap
semiconductor material, its photocatalytic efficiency is lower when the
sunlight was used. Several attempts has been performed to increase its photocatalytic
activity by metal ion doping, noble metal modification, surface enhanced sensitivity and
composite semiconductor. Composite semiconductor is proved an effective technology. To
begin with, in accordance with the IUPAC classification[4,5], based on characteristic
adsorption effects, pores with sizes of 2~50 nm (the diameter of cylindrical pores or the
width of slit pores[5]) are referred to as mesopores. The mesoporous material has
important value of application on macromolecule catalysis , adsorption and separation,
which has the characteristic of high specific area , high porosity and the narrow
distribution of aperture. The composite way of photocatalyst have two kinds, one is
composition[6] and another is coating[7]. In this paper, a series of mesoporous nano-TiO2-SnO2
composite material were synthesized by normal pressure liquid-phase reaction, using
TiCl4 and SnCl4 as raw materials, ammonia liquor as precipitat,
active carbon as templet, and PEG-6000 as anti-agglomerating reagent, and their
photo-catalytic property were also studied.
1 EXPERIMENTAL
Distilled water was used in the whole experiment, and all other chemicals used for the
preparation of mesoporous nano-TiO2-SnO2were of analytical grade.
The characterisition of mesoporous nano-TiO2-SnO2 was performed on
DT-40 thermal analyser, a Y-2000 X-ray diffractometer, a JEM-1000SX transmission
electrical microscope, a ASAP 2020 multiges system, a UV-1200 spectrophotometer and a
KYKY-1000B scanning electronical microscope£¬respectivity.
The synthesis process of nano-TiO2-SnO2mesoporous
material was described as follows:0.1mol TiCl4 were added slowly dropwise into
250mL mixed solution of SnCl4 and PEG-6000, then some active carbon were added
, dispersed about 30 min by ultrasonic. At neutral condition, the obtained solution was
heated rapidly to 100¡æ, and then kept at this
temperature for 1~6 h for hydrolysis of TiCl4 and SnCl4. The
precursor of nano-TiO2-SnO2 was obtained after cooling down to room
temperature, filtering, washing and drying at 100¡æ.
The precursor was air-calcined at 600¡æ for 2 h to
obtain the final product of nano-TiO2-SnO2.
Experiments of adsorption and photo-catalytic degradation were
performed on a UV-1200 spectrophotometer with wavelength from 200 nm to 800 nm. A 200 mL
volume of 50 mg/L acid red 3R dye in a beaker was mixed with 50 mg of product, and then it
was magnetically stirred for 30 min at a dark condition. A comparison between adsorption
valuation of self-made products and Degussa-P25 manufactured by Degussa Corporation
(Germany) was made. Then the beaker was irradiated by sunlight. A fixed amount of sample
was collected from the beaker, and was monitored on a UV-1200 spectrophotometer from 200nm
to 800nm to investigate the photo-catalytic degradation of nano-TiO2 doped with
Sn. The efficiency of degradation was calculated from:
D %= [(A0-A)/ A0] ´ 100%
where A0 and A were the initial absorbance and final absorbance
at the maximum absorption peak, respectively.
2 RESULTS AND DISCUSSION
2.1 Effect of the Sn content
The effect of Sn content in the final product on photo-catalysis was investigated. When Sn
content was below 4 %, the efficiency for degradating acid red 3R dye increased with Sn
content. However, when Sn content was beyond 4 %, the degradation efficiency decreased
with the increase in Sn content, when Sn content was 4 %, the degradation efficiency
reached the maximum (see Table1). The mechanism may be as follows: the conduction band of
SnO2 was about +0.45 eV, and that of TiO2 was about -0.5eV(which was
relatived hydrogen electrode, pH=1). After the pair of electron and hole was produced when
TiO2 excited by ultraviolet light, electron would move to the conduction band
of SnO2, hole would stay on the valence band or be captured, this process would
accelerate the separation of electron and hole, then the photocatalytic efficiency was
improved [8]. But the nanocrystalline TiO2 was gradually transformed from
anatase phase to rutile phase with the increase of Sn-doping, which reduced the
photocatalytic activity of the sample.
Table1.The degradation(D) of acid red 3R
Sn %(mol) |
0 |
2 |
4 |
10 |
20 |
40 |
60 |
80 |
100 |
D % |
94.6 |
96.8 |
100 |
73.4 |
61.2 |
62.3 |
53.5 |
54.7 |
28.4 |
2.2 Effect of the reaction time
The influence of reaction time on yield was investigated under different reaction times.
When the reaction time was shorter than 4 h, the yield was low due to the insufficient
reaction. It was found that the yield reached 96 % in 4 h and particle size was about 20
nm. However, the yield was also decreased with prolonged reaction time, because both
dissolution of tiny crystal particles and continuous growth of large crystal particles may
result in the increase in particle size of the final product (see Fig 1).
2.3 the choice of calcination
temperature
Fig 2 showed the TG-DTA curves of composite powder
precursor that is not calcined. There was a endothermic peak at 64oC in DTA curve, due to
the moisture volatilization adsorbed by media material probably, which resulted in an
obvious the mass loss phenomenon in TG curve. Additionally, two exothermic peaks were in
DTA curve that were at 296.1oC and 582oC, which had the mass loss phenomenon incidentally
in TG curve. This was because PEG-6000 organic molecular was oxidized volatilization at
296.1oC and active carbon was oxidized to CO2 at 582oC. When the temperature
was above 650oC, the weight of the sample was no change, active carbon was completely
converted to CO2. the crystal phase transformation of the nanocrystalline TiO2
from anatase to rutile would happen above 800¡æ,
resulting from the trend of appearing a radiative peak.
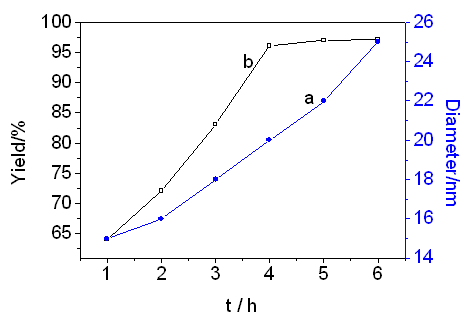
Figure 1 Effect of the reaction
time
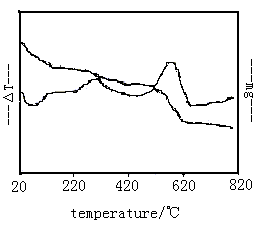
Figure 2 TG-DTA
curve of sample on size(a) and yield(b)
The
granularity of the final product decreased greatly because of the steric hindrance from
PEG-6000 macromolecule, segregation from polymer and anti-agglomeration from active
carbon. Because there were many cavities left after calcinations at 600¡æ for carbon elimination,
nano-mesoporous material with uniform granularity was obtained.
2.4 N2 adsorption-desorption isotherms
The N2 adsorption¨Cdesorption isotherms of the synthesized materials exhibited typical Langmuire ¢ô pattern with the presence of
hysteresis loop as exemplified in Fig 3 for the sample calcined at 600¡æfor 2 h. The hysteresis loop was
typically ascribed to the existence of mesopores in the products. A sharp increase in
adsorption volume of N2 was observed and located in the P/P0 range
of 0.7~1.0.This sharp increase could be assigned to the capillary condensation, indicating
good homogeneity of the sample and fairly small pore size since the P/P0
position of the inflection point was pertained to pore dimension. As illustrated in the
inset of Fig 3, the pore size distribution obtained from normal pressure liquid-phase
reaction was quite narrow, verifying good quality of the samples. Moreover, the isotherms
revealed a single and well-defined step and a clear hysteresis loop in the desorption
branch, pointing out some diffusion bottlenecking in the pore structure.
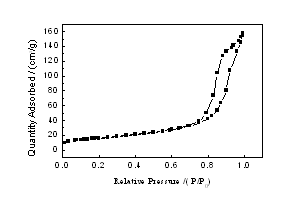
Figure 3 N2 adsorption-desorption isotherms of the sample
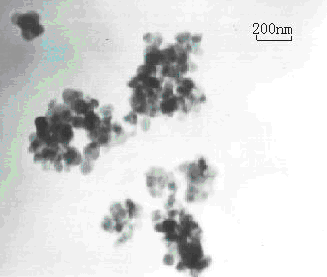
Figure 4 TEM photograph of the sample
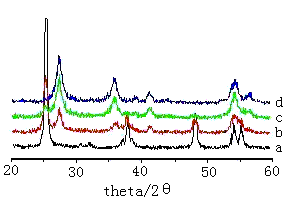
Figure 5 XRD pattern of TiO2
with different Sn-doping
a.0 % b.4 % c.8 % d.10 %
2.5 TEM analysis
TEM analysis of the synthetic samples showed that the diameter of particles were 15~20nm,
there were many pores in the sample, the diameter of which were primary uniform, but the
arrange of which were randomly disorder (see Fig4).
2.6 XRD analysis
A series of nano-TiO2 material doped with different ratios of Sn and Ti were
calcined at 600¡æ. The
transformation temperature of the nanocrystalline TiO2 from anatase phase to
rutile phase decreases with Sn content increasing, when the content of Sn was 10 %, the
crystal phase of the sample was pure rutile (see Fig 5). Anatase structure was composed of
TiO6 octahedron via the same edge, rutile structure was via crank point and
edge, so rutile structure was more stable. SnO2 of similarly rutile structure
would be obtained after calcination at 600¡æ, this was another possible reason that the phase
transform temperature of TiO2 decrease.
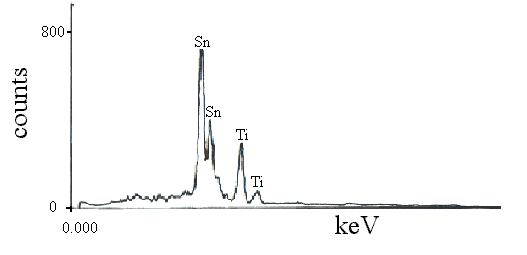
Figure 6 EDS pattern of sample
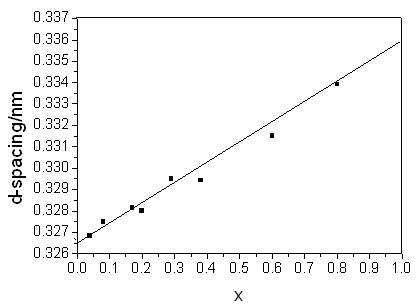
Figure 7 d-spacing-component of
sample
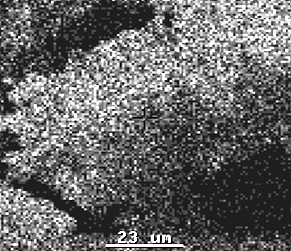
Ti
Sn
Figure 8 Surface distribution of Ti and Sn
2.7 EDS analysis
A series of nano-TiO2 material doped with different ratios of Sn and Ti were
analyzed by EDS. EDS results showed that the ratio of Sn and Ti in the final product was
almost the same as that in the used raw material. Fig 6 was the EDS photograph of the
sample containing 60 at. % Sn. EDS results showed that Sn: 58.28 at. % and Ti: 41.72 at.
%, which was in the range of error permission. The d-spacing increases with increasing
values of x, because the radius of Sn4+ ion (0.071nm£©was larger than that of Ti4+ ion (0.068 nm). Fig7 showed
that the d-spacing (110 plane) linearly increased with x (Sn content), which was
consistent with the Vegards's law[9]. This proved that Sn would enter into square crystal
lattice instead of Ti. As shown in Fig 8, the distribution of Ti and Sn was uniform,
indicating that Sn and Ti were homogenized in the whole product.
2.8 Property of adsorption and photo-catalysis
From Fig 9, it could be clearly seen that the adsorption of nano-TiO2 material
doped with Sn was much better than those of Degussa-P25 and self-made pure TiO2,
the reason would be discussed, the nanometric TiO2-SnO2 compound
mesoporous material had wider specific area prepared by this method, another reason maybe
that the surface acidity of composite oxidant was higher than that of single oxidant,
resulting in enhanced adsorption of the sample [10]. The time of degradation dye was
shorten and the degradation efficiency was improved.
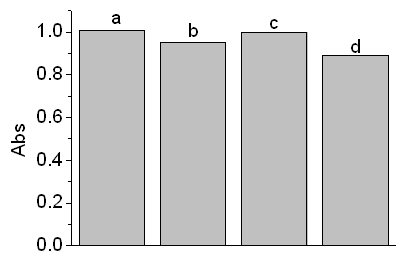
Figure 9 adsorption of acid red 3R
dye
a: no adsorption, b: P25, c:
nm-TiO2, d: nm- TiO2-SnO2
The material had the best
photocatalytic reactivity to acid red 3R when the content of Sn is 4 %, which was the
composite-crystal compound of anatase and rutile. Nanoparticle of different structure in
mixed crystal formed syntopy structure on crystal plane scale, this crystal syntopy would
form transition energy state, which would improve the photocatalytic activity of the
material. Additionally, two kinds of different structure nanoparticle formed similar
doping level due to different energy band width, which would prolong the lifespan of
photoexited electron, improve the utilization rate of sunlight and photocatalytic
efficiency. As shown in Fig 10, the spectra indicated that absorption intensity for acid
red 3R dye solution decreased gradually with prolonging photo-catalytic time, and when
photo-catalytic time was beyond 40 min, no apparent absorption was observed and
decolorization rate was considered as 100 %. (This was supported by the results from K2Cr2O7
method).
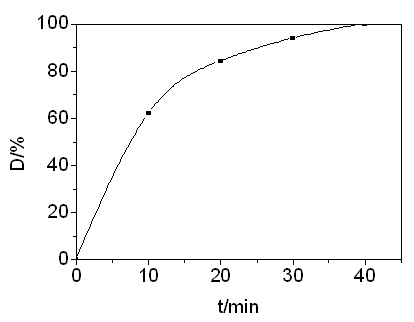
Figure 10 Degradation curve of acid red 3R
dye
3 CONCLUSIONS
Using ordinary inorganic salt as
raw materials, and active carbon as template, utilizing the advantages of short reaction
time, operation convenience and low energy loss with normal pressure liquid-phase method
and steric hindrance effect of PEG-6000 macromolecule, nano-TiO2-SnO2
mesoporous material was prepared . The sample has uniform granularity and sensitivity to
UV and visible light.
Experimental results of
optical adsorption and photo-catalysis showed that the sample has best photo-activity when
the concent of Sn is 4 %, which is partly transformation from anatase phase to rutile
phase and the degradation rate of acid red 3R dye solution can be
up to 100 % because of the triplicate synergism effect.
Acknowlegements This work was supported
by the Natural Science Foundation of Hebei Province, China (Grant No.200500097)
REFERENCES
[1] ZHANG Li-De,MOU Ji-Mei Nano Material and Nano-Structure,Beijing: Science
Press,2001,p59,p88,p90.
[2] HE Bei-Ping,WANG Zhan-Sheng et al Huanjing Kexue(Chinese Envion.Sci.),1994, 15(3),80.
[3] Yoneyama H. Cryt. Rev. Solid State Mater.
Sci., 1993,p18,p69.¡¡
[4] IUPAC Manual of Symbols and Terminology, Appendix 2, Pt.,
Colloid and Surface Chemistry, Pure Appl. Chem. 31 (1972) 578
[5] S.J. Gregg, K.S.W. Sing, Adsorption, Sruface Area and Porosity, Academic Press,
Lomdon, 2nd edn., 1982, p.93
[6] Vinodgopal K,Kamat P V. Environ. Sci.&Technol,1995,29:841
[7] Shi L Y,Gu HC,Li CZ, et al. Chinese J.Catal,1999,20:338
[8] Liu Ping,Zhou Ting-Yun,et al.Acta
Phys.-Chin.Sin.,2001,17(3):265-269¡¡
[9] West , A. R. ,Solid State Chemistry And Its Applications,
New Delhi: John Wiley, 1984, 367, 366~370
[10] Fu X,Clark L A,Yang Q,et al. Environ.Sci,& Technol.,1996,30:647¡¡
¡¡
|