Reduction of second-order scattering
interference by variable-angle synchronous luminescence spectroscopy
Li Yaoqun, Qian Fang, Li Zhao
(Department of Chemistry and Key Laboratory of Analytical Sciences of MOE, Xiamen
University, Xiamen 361005, China)
Received Feb. 10, 2000; Supported by the
National Natural Science Foundation of China.
Abstract A means of reducing the
interference of second-order Rayleigh scattering is proposed by using variable-angle
synchronous luminescence (VASL) spectra. This approach involves keeping a VASL scanning
route parallel to the second-order scattering trajectory in the excitation and emission
matrix. The principle of reducing the interference is described and the examples of water
blank and a porphyrin molecule confirm the assumption. Derivative technique is suggested
to couple VASL to reduce the second-order scattering while the fluorescence of a molecule
and the scattering of solvent overlap seriously. Furthermore, it was suggested that
variable-angle synchronous scanning can be used to plot directly a second-order scattering
spectrum when the spectrum itself is utilized.
Keywords Variable-angle synchronous luminescence spectra, Rayleigh scattering,
second-order scattering light, fluorimetry
It has been known that various scattering
light, including Rayleigh scattering, Tyndall scattering and reflected light and Raman
scattering, can distort the excitation and emission spectra in low concentrations and
impede the highly-sensitive measurements and identifications for fluorescent compounds.
When the emission wavelength of a fluorescent molecule is far from the excitation
wavelength, second-order scattering light should be paid attention to. This situation may
happen more frequently when a red-region or near-infrared fluorescent dye is used. In
cases in which the fluorescence or phosphorescence characteristics of a molecule are
unknown, the signal of scattering light may even be mistakenly identified as analyte
emission. Therefore, avoiding or eliminating various signals of scattering light is often
a key procedure for increasing the linear dynamic concentration ranges, improving
detection sensitivity and obtaining a correct spectrum in fluorescence and phosphorescence
analyses. Time-resolved detection, the use of filters and synchronous luminescence
spectroscopy provide different approaches to reduce the influences of scattering light.
Synchronous luminescence spectroscopy has the merits of simplifying
spectral complexity, improving selectivity and decreasing scattering light interference,
and has been found more and more applications in various fields of environmental science,
medicine, pharmacology and hydrology. This technique has been developed into three
variants. Conventional constant-wavelength synchronous luminescence spectroscopy (CWSLS)[1-5]
has been used to eliminate the first-order interference of Rayleigh scattering, Tyndall
scattering, light reflected off the cell faces, while constant-energy synchronous
luminescence spectroscopy (CESLS)[6-10] has been used to eliminate the
interference of Raman scattering.
Variable-angle synchronous luminescence spectroscopy (VASLS)[4,11-14]
provides a most flexible technique in selecting experimental parameters among three
variants of synchronous spectroscopy. In this paper, an approach of suppressing the
interference of second-order scattering light by using VASLS is proposed and the coupling
of derivative technique is further suggested to overcome the limit of VASLS. The method
and the examples of eliminating second-order scattering light are presented.
1. THEORY
Rayleigh scattering is usually in majority for a solution among those interference factors
that occur at the emission wavelength equal to the excitation wavelength. Considering the
existence of two monochromators (excitation monochromator and emission
monochromator) for a spectrofluorimeter, actually there are two kinds of second-order
Rayleigh scattering in fluorimetry. One is the second-order rays of Rayleigh scattering
and the other is the Rayleigh scattering of second-order rays. The former appears at the
emission wavelength twice as long as the excitation wavelength (lem =2lex). The latter appears at the emission wavelength half
as long as the excitation wavelength (lex =2lem). Since fluorescence emission would not exist at the
wavelength region shorter than excitation (except two-photon fluorescence), we will not
pay attention to the latter scattering in this paper.
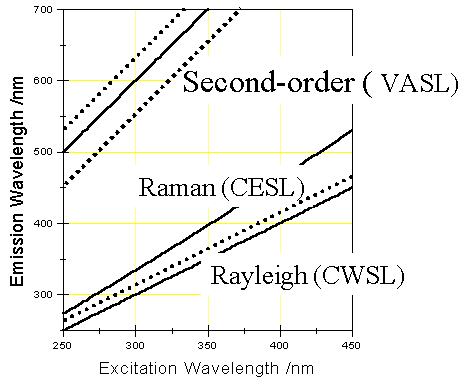
Fig. 1 Schematic diagram of trajectories of Rayleigh scattering, Raman scattering
and second-order Rayleigh scattering in EEM. The scatterings can be avoided with the CWSL,
CESL, and VASL techniques, respectively, by keeping a scanning route parallel to
scattering trajectories (dot lines).
The schematic trajectories in the excitation and emission matrix (EEM)
shown in Fig. 1 depict different scattering lights and their reduction by different
scanning approaches. CWSLS is capable of eliminating the first-order Rayleigh scattering
interference by scanning parallel to Rayleigh scattering ridge. CESLS maintains a
constant-energy difference Dn between the excitation and emission monochromators while scanning.
By keeping a Dn different
from Raman scattering energy Dn R, Raman scattering does not appear in CESL spectra.
The way of the elimination of second-order scattering light is similar to the above. VASLS
can make full use of excitation and emission information of molecules by synchronously
scanning excitation and emission monochromators at different speeds. Based on the
principle of the VASL scan, the following equation can be obtained for a VASL scan path[4].
lem=b(lex-lex*)+glex*
(1)
where b
is the ratio of the scan speed for emission monochromator to the one for the excitation
monochromator or the slope of a VASL path across an EEM. lex* is the initial wavelength positions for
excitation monochromator and g is the ratio of the initial emission wavelength to the initial
excitation wavelength. Thus, b and g are the two key parameters in defining a VASL scan path.
It can be supposed that if the emission wavelength keeps twice the
excitation wavelength during wavelength scanning, i.e. b = g=2, the scan path will run along the second-order scattering light and
second-order scattering spectra will be obtained. If the scan path runs parallel to the
second-order scattering path ( b =2 and g ¹ 2), the influence of second-order
scattering will be eliminated. VASLS is the only technique that is able to meet the
requirement of scanning such a path. So long as the wavelength distance between the
selected VASL path and the second-order scattering path is kept far enough, the VASF
spectrum would not have second-order scattering interference. Therefore, VASLS can be used
to eliminate the influence of second-order scattering in a spectrum.
2. EXPERIMENTAL
All spectra were conducted with a laboratory-constructed MYF spectrofluorimeter equipped
with a 500-W xenon lamp and excitation and emission grating monochromators[8,10,14].
This apparatus can be used for measurements of fluorescence excitation spectra, emission
spectra, all kinds of synchronous spectra (including CW, CE and VA modes) and their
derivative spectra. To obtain scattering-suppressing VASL spectra, the scan speed of
emission wavelength is kept twice that of excitation wavelength and the initial emission
wavelength is set different with twice the excitation wavelength.
Meso-tetra(4-sulfnatophenyl)porphine (TPPS4) (Porphyrin
Products, INC) was used without further purification. TPPS4 solutions were
prepared in water. Deionized and distilled water was used.
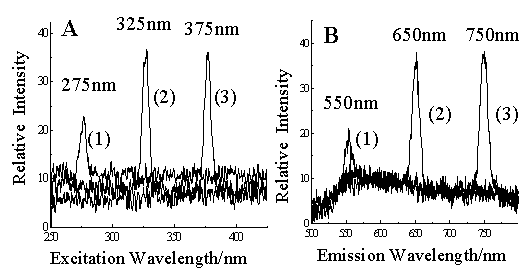
Fig. 2 Blank excitation and emission
spectra of water.
A. Excitation spectra obtained with the emission wavelength at 550 nm (curve 1), 650 nm
(curve 2), and 750 nm (curve 3);
B. Emission spectra obtained with the excitation wavelength at 275 nm (curve 1), 325 nm
(curve 2), and 375 nm (curve 3).
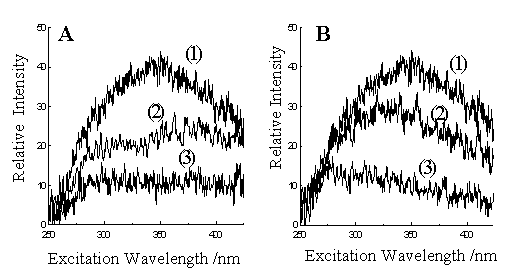
Fig. 3 Blank VASL spectra of water. All experimental conditions are
the same except the g values ( b=2 for all
spectra):
A. g=2 (curve 1), 1.96 (curve 2),
1.94 (curve 3);
B. g=2 (curve 1), 2.02 (curve 2),
2.06 (curve 3).
B(1) is the same spectrum as B(2) and is re-plotted for comparison.
3. RESULTS AND DISCUSSION
Fig. 2 shows the blank excitation and emission spectra for water. The
peaks at 275, 325, 375 nm in the blank excitation spectra respectively were second-order
scattering ones with the emission wavelength set at 550, 650, 750 nm respectively for each
excitation spectrum (Fig. 2A). The second-order scattering peaks in the blank emission
spectra appeared at 550, 650, 750 nm respectively when using the excitation wavelengths at
275, 325, 375 nm respectively (Fig. 2B). Second-order Rayleigh scattering of water
appeared when the emission wavelength twice the excitation wavelength. Actually the peak
at 275 nm in the excitation spectra corresponds to the peak at 550 nm in the emission
spectra. Though scattering light may be out of the fluorescent spectral range of a
specific compound, it is unavoidable to have the second-order peaks in excitation or
emission spectra.
Several blank VASL spectra of water are shown in Fig. 3, which
illustrate the effect of VASL scan parameters on the blank spectra. All spectra were
obtained at identical experimental conditions except different variable-angle synchronous
scan routes. The scanning speed of the emission monochromator is kept to be twice as fast
as that of excitation monochromator for all these spectra (b =2). The ratio g of the initial emission wavelength to the initial excitation
wavelength was varied. For those spectra shown in Fig. 3, the initial excitation
wavelength was maintained at 250 nm and the emission wavelength was varied. When g was equal to 2, the worst blank VASL
spectrum was obtained as shown in Fig. 3A(1) (or Fig. 3B(1)). Actually it was just the
second-order scattering spectrum of water since the scan path is along the second-order
scattering trajectory. The maximum of the second-order scattering light appeared at VASL
spectrum was ca. 350 nm, which was the result of both the intensity variance of the
second-order scattering and the factor of instrumental response. When g was different from 2, the second-order
scattering signal becomes weaker rapidly as seen from Fig. 3. In Fig. 3A, the middle
spectrum was obtained at g
equal to 1.96 and the bottom spectrum at g equal to 1.94. In Fig. 3B, the middle spectrum was obtained at g equal to 2.02, and the bottom spectrum
at g equal to 2.06. When g was larger than 2.06 or smaller than
1.94, the second-order scattering signals of water became very weak. Thus, by selecting a
suitable variable-angle synchronous scanning route, second-order scattering can be
eliminated.
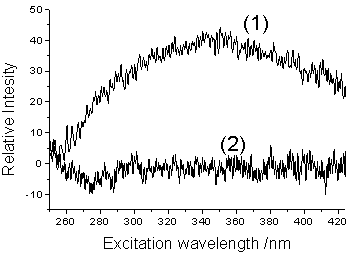
Fig. 4 Blank zero-order (1) and first-order (2) derivative VASL
spectra of water. Spectrum (1) is actually the same as Fig. 3A(1). Both b and g were set at 2 for the VASL scan
route.
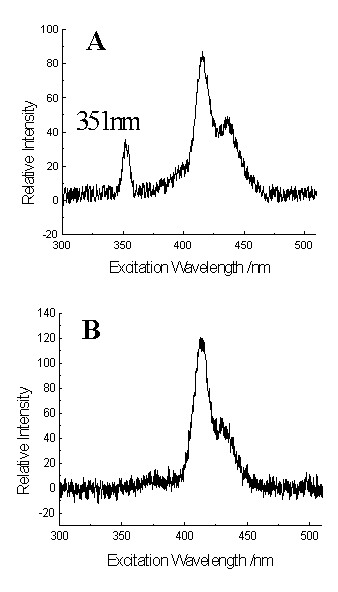
Fig. 5 Comparison of conventional and VASL spectra of
TPPS4 (10-7 mol/l).
A. Fluorescence excitation spectrum obtained with the emission wavelength at 702 nm.
B. VASL spectrum obtained with b=2 and g=1.43
It is also possible to perform a fluorescence measurement using VASL
scanning along the second-order scattering ridge (b=2 and g= 2) or nearby (g ranging from 1.94 to 2.06) by combining derivative technique.
Usually the second-order scattering ridge should be avoided. However, for those compounds
with fluorescence emission approximately at the wavelength twice the excitation
wavelength, this route is needed to obtain intense fluorescence and good selectivity.
Derivative technique has the advantage of suppressing broad band and enhancing weak but
sharp peak or shoulder. It has been used to improve spectral resolution in VASLS[12,14]
and to reduce Raman scattering in CESLS [10]. Derivative technique can be
particular useful for reducing the interference of the broad second-order scattering band.
Fig. 4 is the derivative VASL spectra of water when b=2 and g= 2. The broad-band intense spectrum of
scattering became nearly-zero after derivation. Thus, derivative
technique can be coupled with VASLS to overcome further the limit of VASL approach in
reducing second-order Rayleigh scattering interference.
TPPS4 was used to illustrate further the method to eliminate the
second-order scattering peaks (Fig. 5). TPPS4 has two emission maximum at 646
and 702 nm. When using 702 nm as the emission wavelength, a second-order scattering peak
at 351 nm can be observed in the excitation spectrum (Fig. 5A), which is close to the
fluorescent excitation range of the compound. For traces of TPPS4, the
contribution from scattering light became appreciable so that it is important to eliminate
the scattering interference while working in low concentrations to avoid the spectral
distortion. However, there is not such a peak of second-order scattering in VASL spectrum
(Fig. 5B). The scan speed of the excitation monochromator was 120 nm/min and that of the
emission monochromator is 240 nm/min. The initial excitation wavelength of 300 nm and the
initial emission wavelength of 430 nm were applied. This suppressing of second-order
scattering was not at the cost of sensitivity. The relative peak intensities obtained from
fluorescence excitation (Fig. 5A) and VASL (Fig. 5B) spectra were 87 and 118,
respectively, which indicated a slight better sensitivity for the latter.
In summary, we have proposed a technique to reduce second-order
scattering interference by selecting a suitable VASL scanning route. To eliminate further
the scattering interference, the coupling with derivative technique is also suggested.
What we wish to add to the end of the paper is that the VASL technique
can be used to investigate the second-order scattering light itself since a second-order
scattering spectrum can be simply and easily obtained by the technique. In this paper, the
main target is the elimination of second-order scattering light. For those work on the
utilities of scattering spectrum [15-16], first-order scattering spectra have
been usually obtained from a spectrofluorimeter with constant-wavelength synchronous
scanning (Dl = 0 nm); second-order scattering spectra have been only re-plotted
from separate data of measurement, which is a trouble and time-consuming procedure. A second-order
scattering spectrum (see Fig. 3B(1)) can be plotted directly by variable-angle synchronous
scanning with b=2 and g=2.
REFERENCES
[1] Vo-Dinh T, Fetzer J, Campiglia A D. Talanta, 1998, 47: 943.
[2] Ruiz T P, Lozano C M, Tomas V, Carpena J. Talanta, 1998, 47: 537.
[3] Lopez M H, Gonzalez M A, Molina Ma I L. Talanta, 1999: 49: 679.
[4] Li Y Q, Huang X Z. Fresenius J. Anal. Chem., 1997, 357: 1072.
[5] Li Y Q, Huang X Z, Xu J G. J. Fluorescence, 1999, 9 (3): 173.
[6] Inman E L, Winefordner J D. Anal. Chim. Acta, 1982, 138: 245.
[7] Miller J S. Anal. Chim. Acta, 1999, 388: 27.
[8] Li Y Q, Shi N, Qian F, Huang X Z. Chem. J. Chin. Univ. (Gaodeng
Xuexiao Huaxue Xuebao), 1997, 18: 538.
[9] Andre J C, Bouchy A, Jezequel J Y. Anal. Chim. Acta, 1986, 185: 91.
[10] Li Y Q, Huang X Z, Chen G Z. Chin. Sci. Bull. (Kexue Tongbao),
1991, 36: 1312.
[11] Carretero A S, Blanco C C, Gutiérrez A F. Anal. Chim. Acta, 1997, 353: 337.
[12] Pulgarin J A M, Bermejo L F G. Anal. Chim. Acta, 1998, 373: 119.
[13] Carretero A S, Blanco C C, Gutierrez A F. Analyst, 1997, 122: 925.
[14] Li Y Q, Huang X Z, Xu J G. Anal. Chim. Acta, 1993, 283: 903.
[15] Li Y F, Huang C Z, Hu X L. Chin. J. Anal. Chem. (Fenxi Huaxue),
1998, 26 (12): 1508.
[16] Liu S P, Liu Z F, Li M. Acta Chim. Sinica (Huaxue Xuebao),
1995, 53: 1185.
|