Framework collapse process
of silicoaluminophosphate molecular sieve at high temperature by 27Al multiple
quantum and multi-nuclear MAS NMR
Chen Tiehong*,#, Wouters B
H#, Grobet P J #
(*Department of Chemistry, Nankai Univerisity, Tianjin, 300071; #Center
for Surface Chemistry and Catalysis, Katholieke Universiteit Leuven, Kardinaal Mercierlaan
92, B-3001 Heverlee (Leuven), Belgium )
Received Aug.14, 2000; Part of this work was
supported by the National Natural Science Foundation of China (Grant No. 29803004)
Abstract Molecular sieve SAPO-37
has been studied by 27Al MQMAS and multi-nuclear solid state NMR methods. It is
proved that in the dehydrated state, the aluminum signal of the Bronsted sites in SAPO-37
is visible, and tri-coordinated Al may cause intensity loss due to the quadrupolar
broadening. When calcined at 1173 K, Si atoms become mobile and detach from the framework
to aggregate to form polymorph silica, while an Al-P dense phase was formed, corresponding
to a new Al signal which is distinguished by the 27Al MQMAS spectrum for the
first time. The move of atoms in SAPO-37 at 1173 K is the start of the collapse of the
framework.
Keywords molecular sieve SAPO-37, 27Al MQMAS NMR, polymorph silica
Silicoaluminophosphate molecular sieve
SAPO-37, an isotype of faujasite, has been the subject of numerous researches on the study
of mechanism of silicon substitution, the silicon environments and their catalytic
properties [1-6]. A drastic change of the 29Si MAS NMR spectrum in
SAPO-37 after heating at 1173K was observed and a model of "solid state
transformation" was proposed to explain the
environment change of Si atoms calcined at the temperature between 1073-1173K in SAPO-37 [7,8];
this model involves the floating of atoms in the framework lattice to form the
siliceous islands and silicoaluminate domains, while at the same time, the faujasite
crystal structure is maintained. An increase of the catalytic activity of SAPO-37 calcined
at 1173 K was explained by the newly formed silicoaluminate phase during the "solid
state transformation".
Although the 29Si NMR spectrum shows different Si
environments (Si with different numbers of Al as next nearest neighbors and Si islands),
the 1D 27Al MAS NMR spectra could not be well resolved due to the quadrupolar
broadening of aluminum nucleus. 27Al Multiple Quantum (MQ) MAS NMR method [9,10],
which can refocus the second-order quadrupolar broadening, has been used to study the
SAPO-37 and an enhanced resolution was obtained, and the Al environments with and without
Si as the nearest neighbours has been distinguished [11]. Here by means of
29Si, 27Al, 31P, 1H MAS NMR, together
with 27Al MQ MAS NMR methods, we examined the thermal stability and structure
change of the SAPO-37 after high temperature calcination.
1 EXPERIMENTAL
A SAPO-37 sample was synthesized hydrothermally [12] with the composition of
[Si23Al98P78O384]:TMA8:TPA12:64H2O
as determined by electron probe microanalysis and thermal analysis. The XRD spectrum
demonstrates the sample is highly crystalline. The 29Si MAS NMR spectrum shows
isolated Si (pure SAPO phase) as well as a small amount of Si islands in this sample.
The template-free sample was obtained by heating the as-synthesized
sample at 873 K in a quartz tube in a flow of O2 for 20 hrs, with temperature
increment of 1 K/minute. And the samples were calcined at 1073 or 1173 K for 10 hrs in a
quartz tube in vacuum respectively. Because H-SAPO-37 is very sensitive to water, after
the thermal treatment the quartz tube was closed and transferred into a glove box with dry
N2 where a 4 mm rotor was packed.
The 27Al and 31P MAS NMR experiments were
performed on a Bruker MSL-400 spectrometer at 104.26 MHz and 161.5 MHz, respectively, with
a 4 mm ZrO2 rotor spinning at 12.5 kHz. The 31P chemical shift is
referenced to 85% H3PO4. The 1D 27Al MAS NMR were
performed with a pulse length of 0.6 m s corresponding to a p/18 pulse length and a recycle delay of 0.1 s. A two-pulse 3Q MAS
method [10] was employed with pulse lengths of 2.7 and 0.8 m s respectively, a radio
frequency field of ca. 120 kHz and a 0.5 s recycle time; 512 points were acquired in the t1
dimension with increments of 6 m s; 480 scans were recorded per FID. Chemical shift of F2
dimension is referenced to an Al(NO3)3 solution.
1H NMR was performed on a Bruker AMX-300 spectrometer at
300.13 MHz, with a spin rate of 10 kHz and 10s recycle delay with a p/2 pulse length of 3.5 ms. 29Si NMR was performed
also on a Bruker AMX-300 spectrometer at 79.5 MHz, with a spin rate of 4 kHz and 10 s
recycle delay. The 1H and 29Si chemical shift was referenced to
tetramethylsilane (TMS).
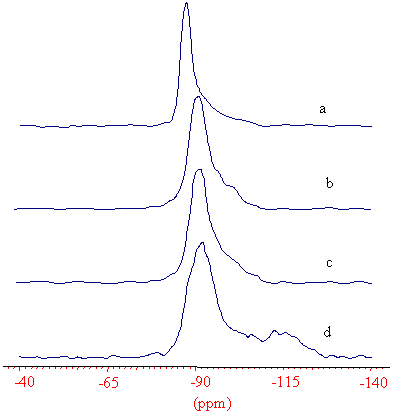
Fig. 1 29Si MAS NMR spectra of the
(a) as-synthesized, (b) 873, (c) 1073, (d) 1173 K calcined SAPO-37
2 RESULTS AND DISCUSSION
The 29Si MAS NMR spectra of as-synthesised, 873, 1073, 1173 K calcined SAPO-37
are displayed in Fig. 1. The -89 ppm in the
as-synthesised sample is the isolated Si(4Al), and a small up-field hump corresponding to
small amount of Si islands [2]. In the spectrum of the 873 K calcined samples (Fig 1b),
the signal of isolated Si shifts to -92 ppm and become
broader due to the burnt-out of the templates. There is no much difference displayed in
the 29Si NMR spectra of the 873 and 1073 K calcined samples (Fig.1b,c), though
some dehydroxylation takes place in the later sample (as observed by the 1H NMR
measurement). The 29Si MAS NMR spectrum of the 1173K calcined (Fig.1d) looks
quite different, a broad hump emerges: a -115 ppm signal can be distinguished and some
signals in between -115 and the -92 ppm, but could not
be resolved due to their wide chemical shift dispersion. According to the literature [8,
13], the -115 ppm signal can be assigned to the
polymorph silica structure, for instance, the tridymite. This single can also be seen in
the Peltre's results [7], in which the change of the 29Si MAS NMR
spectrum of the SAPO-37 calcined at 1173 K was reported. This newly formed structure can
be identified by the XRD spectrum that an additional peak of tridymite, supporting the
assignment. It is a clear evidence that when calcined at 1173 K, some topology phase
transformation takes place in SAPO-37.
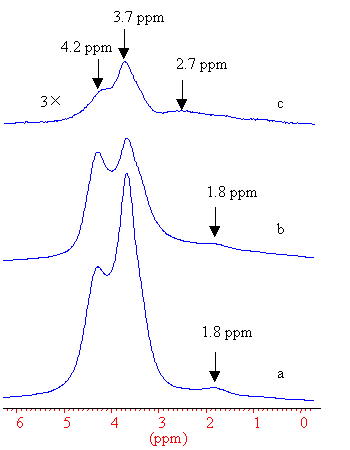
Fig. 2 1H MAS NMR spectra of (a) 873, (b) 1073,
(c) 1173 K calcined SAPO-37
The 1H MAS
NMR spectra were shown in Figure 2. The 1.8 ppm was assigned to the silanol group, and 3.7
and 4.2 ppm were assigned to the bridging hydroxyls in the supercages and sodalite cages,
respectively [14]. By the comparison of the 1H NMR signal
intensities, it can be concluded that dehydroxylation takes place when calcined at 1073 K,
and the hydroxyls in the supercage are detached firstly. When the sample is calcined at
1173 K, most of the hydroxyls are removed, and a signal at 2.6 ppm emerges which could be
assigned to the extraframework Al-OH, indicating that some dealumination takes place at
this high temperature.
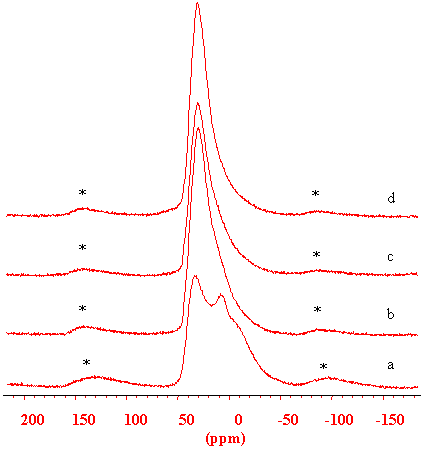
Fig.3 27Al MAS NMR spectra of the (a)
as-synthesized, (b) 873, (c) 1073, (d) 1173 K calcined SAPO-37, * indicate the spinning
side bands.
27 Al MAS NMR
spectra of the as-synthesised, 873, 1073, 1173 K calcined samples are displayed in Figure
3. In the study of zeolites, 27Al NMR measurement is difficult to perform on
the dehydrated samples due to the very strong quadrupolar interaction of the aluminum, and
there is always intensity loss [15]. This it is not the case in the SAPO-37
system. By quantitative intensity comparison it is shown that there is no 27Al
NMR intensity loss in the spectrum of the 873 K calcined sample compared with the
as-synthesized sample, i.e., in the dehydrated H-SAPO-37, the Al (nSi) environment is
completely detectable. It is obviously due to the chemical composition of the SAPO system
with the presence of the phosphorus in the balanced framework.
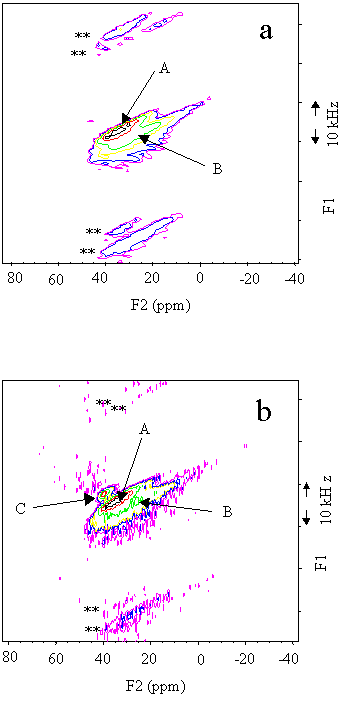
Fig. 4 27Al 2D 3Q MAS
NMR spectra of the (a) 1073, (b) 1173 K calcined SAPO-37; ** indicate the spinning side
bands, A,B,C see text.
The 27Al
intensity of 1073 K calcined sample shows an intensity loss of 15%, due to the formation
of the tri-coordinated Al by dehydroxylation. Although further dehydroxylation continues,
it is very interesting to note that in the 1173 K calcined sample, the Al intensity is
recovered to 95%, i. e., some invisible Al become visible again. This is hardly due to the
recovery of the tri-coordinated Al to its original configuration because the
dehydroxylation continues at higher temperature, but to some further configuration change
of the"invisible" part of the Al. The peak
position of 27Al NMR line of the 873 and 1073 K (Fig 3b,c) calcined samples
moves gradually up-field, probably due to the increasement of the quadrupolar interaction.
For the 1173 K calcined sample (Fig 3d), the peak goes back downfield to 37 ppm, in
agreement with Derewinski et al. [8], and it is postulated that some new Al
species are emerging, whose environment is more symmetric (the quadrupolar effect is
smaller ) to give rise to a downfield contribution beside the framework Al(4P) signal.
To enhance the resolution, 27Al triple quantum NMR method
was employed here. It has been reported that in the as-synthesised SAPO-37, there are
different Al coordinatons with different templates, and in the calcined sample, only two
Al (Al(4P) and Al(Si, 3P)) were identified [11]. In Figure 4a, the 27Al
3Q MAS of 1073 K calcined displayed only two parts, similar to the spectrum of the 873 K
calcined one[11], which could be assigned to the Al(4P) phase and Al(Si, 3P)
environments ( A and B as indicated in the Figure 4, respectively). In the 27Al
3Q MAS NMR spectrum of the 1173 K calcined sample (Fig 4b) it shows that a new Al phase
emerging (indicated as C in the Figure) at 35.9 ppm on F2 and 35.6 ppm on F1, its
quadrupolar constant could be calculated to be about 1 MHz by the formula in reference 16.
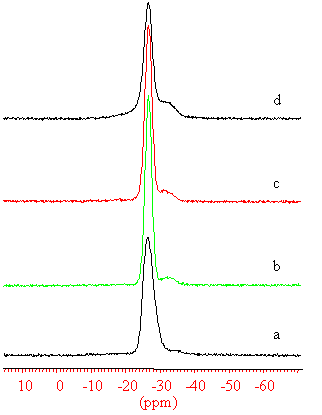
Fig. 5 31P MAS NMR spectra of the (a)
as-synthesized, (b) 873, (c) 1073, (d) 1173 K calcined SAPO-37
This newly formed new
Al phase could result in the chemical shift of the Al(4P) peak to lowfield and the
intensity re-gain due to the reduction of the quadrupolar interaction. By its position
this newly formed Al phase should also be tetrahedral coordinated. Due to its rather
symmetric environment, it is either due to the Al with 4P or 4Si as the nearest neighbors.
By our experiments, the 3Q MAS NMR spectra of the higher Si content SAPO-37, which
contains the Al(4Si) structure units, did not resolve the Al(4Si) environment as a third
signal. Thus we reasonably assign the newly formed Al phase to the Al with 4P
environments; because it can be distinguished from the framework Al(4P) units of the
SAPO-37, its configuration should be different, i.e., it can not be regarded as in the
topology of the faujasite, but due to some dense phase.
The 31P NMR spectra of the as-synthesized, 873, 1073, 1173 K
calcined samples are showed in Figure 5. For the as-synthesised sample, a peak at around -26.2 ppm represents the P(4Al) environment, and small hump at -33 ppm can be assigned to the some defect sites in the framework.
The spectra of the 873 and 1073 K calcined samples display narrower line width (300 Hz) of
the -26.5 ppm P(4Al) signal than that of the
as-synthesized sample (510 Hz), due to the removing of the templates. But the spectrum of
the 1173 K calcined sample became broader again (460 Hz), and there appeared a broader
hump; a wide dispersion of environment of the 31P NMR line of the 1173 K
calcined sample, gives evidence that part of the P were involved in the framework change
at high temperature, together with the change of the Al and the Si environment, as
indicated by the NMR results.
Combining the multi-nuclear NMR results above, it could be postulated
that when calcined at 1173 K, some part of the Al-Si connection in the SAPO-37 framework
break and new Al-P phase form, distinguished as a new separate signal by the 27Al
MQMAS spectrum; the broadening of the 31P NMR signal corresponds to this
framework structure modification. Some Si may be detached from the lattice, as Al does in
the zeolites framework, to recrystallize at high temperature as to form polymorphs.
By our experiments, the SAPO-37 is not thermally stable as it was
regarded. When calcined at 1173K, the framework atoms are in high mobility, and it could
be the start stage of the collapse of the topology. The defect sites during the process of
the framework change may be responsible for the catalytic properties at high temperature.
More experiments on other SAPO systems are needed to see whether the
"de-siliceous" effect is universal in the
SAPO systems.
3 CONCLUSION
Silicoaluminophosphate molecular sieve SAPO-37 has been studied with 27Al MQMAS
and multi-nuclear solid state NMR methods. It is proved that the Al of the Bronsted sites
in SAPO-37 is visible, and tri-coordinated Al may cause intensity loss due to the
quadrupolar interaction. When calcined at 1173 K, Si atoms become mobile and detach from
the framework to aggregate to form polymorph silica, while Al-P dense phase was formed,
corresponding to a new Al signal which can be distinguished by the 27Al MQMAS
spectrum. At 1173 K the new dense phase of Al-P form and the new Si signals can be
explained as extraframework silica phases. The move of atoms in SAPO-37 is the start of
the collapse of the framework.
REFERENCES
[1] Lok B M, Messina C A, Patton R L et al. J.Am. Chem. Soc., 1984, 160: 6092.
[2] Martens J A, Janssens C, Grobet P J et al. Stud. Surf. Sci. Catal., 1989, 49A: 215.
[3] Blackwell C S, Patton R L. J. Phys. Chem., 1988, 92: 3965.
[4] Derewinski M, Briend M, Peltre M J et al. J.Phys.Chem., 1993, 97: 13370.
[5] Su B L, Barthomeuf D. J. Catal., 1993, 139: 81.
[6] Su B L, Barthomeuf D. Zeolites, 1993, 13: 626.
[7] Peltre M J, Man P P, Briend M et al. Catal. Lett., 1992,16: 123.
[8] Derewinski M, Peltre M J, Briend M et al. J.Chem.Soc., Faraday Trans., 1993, 89: 1823.
[9] Frydman L, Harwood J S. J. Am. Chem. Soc., 1995, 117: 5317.
[10] Fernandez C, Amoureux J. Chem. Phys. Lett., 1995, 449: 242.
[11] Chen T H, Wouters B H, Grobet P J. Colloids and Surfaces, A, 1999, 158:145.
[12] Kovalakova M. Ph.D. thesis, Katholieke Universiteit Leuven, 1996.
[13] Briend M, Peltre M J, Massiani P et al. Stud. Surf. Sci. Catal., 1994, 84: 613.
[14] Hunger M, Anderson M W, Ojo A et al. Microporous Mater., 1993, 1: 17.
[15] Freude D, Ernst H, Wolf I, Solid State Nucl. Magn. Reson., 1994, 3: 271.
[16] Rocha J, Esculcas A, Fernandez C et al. J. Phys. Chem., 1996, 100: 17889.
|