Three-distribution-parameter general model
for waste minimization targeted reactor network synthesis
Hua Kailing, Li Yourun, Hu Shanying, Shen
Jingzhu
(Department of Chemical Engineering, Tsinghua University, Beijing, 100084)
Received Oct. 20, 2000; Supported by the National Natural Science Foundation of China
(29836140).
Abstract Based on the
three-distribution-parameter general model for reactor network synthesis proposed earlier
by the author, waste minimization target is included to realize source reduction in
reactor network. The profit maximization design with waste minimization target can be
formulated as a multi-objective optimization problem and e constraint technique is applied to convert it into a single
objective optimization problem. After optimization, not only the reactor type and
configurations can be directly decided according to the optimal solution of distribution
parameters, but also the non-inferior curve can be attained. Two examples are studied and
discussed.
Keywords three-distribution-parameter general model, reactor network synthesis,
waste minimization
Reaction system is the most crucial part in
the whole process integration for profit benefit and waste minimization. Due to the
inherent complexity of the problem itself, the development of reactor network synthesis is
still insufficient. Strategies exploited are chiefly heuristic and mathematical
optimization approach. The later is more fitted in solving large-scale problems and become
the main strategy.
Two main branches of mathematical optimization approaches are
superstructure approach and targeting approach. Superstructure approach is to propose an
initial superstructure and to find a subsystem that produces the best result. Its
advantages include the easiness in adding additional constraints and modifying objectives
in the optimization calculation and the ability of simultaneous achievement of optimal
objective and network. But global optimal solution is always available. Targeting approach
is originated from attainable region concept suggested by Horn in 1964. Ever since then,
two main branches have been developed: geometric approach and constructive targeting
approach. Geometric region approach can provide physical insight into the problem to be
studied but has serious dimension limitation. In constructive targeting, no initial
networks are predetermined and instead a bound on the performance index is tried to find
out. The optimal solutions are formed during a series calculation procedure.
In this paper, based on the three-distribution-parameter general model
proposed in an earlier paper (Hua et. al, 2000), multi-objective optimization strategy is
exploited for the developed optimization framework to solve the waste minimization
problem. Two case studies will be demonstrated on this approach.
1. GENERAL MODEL BASED OPTIMIZATION
APPROACH
1.1 New general model for reactor network synthesis
The importance of the influence of concentration profile and residence time
distribution on reaction results demands on appropriate controlling ways. Three flow
streams are chosen to define the macro mixing state and the connection ways in the reactor
network. Each of them represents some specific characteristic of reactor network and
further more give the comprehensive reflection of optimal network. For example, the side
feeding strategy has great impact on concentration and resident time distribution; the
recycling can represent different back mixing extent so as to decide the reactor type and
the bypassing represents resident time distribution in the network and realizes parallel
structure. But the boundaries between them are not clearly cut and they all can
effectively increase conversion and reduce wastes. So the general model represented by
them is capable of efficient expression for optimal concentration profile and residence
time distribution. Figure 1 shows a schematic diagram for this general model. And it can
also be called DRDSR, which is differential-recycling DSR.
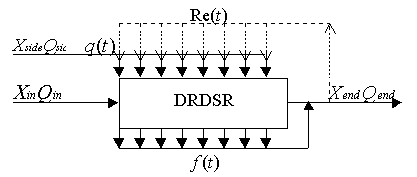
Figure 1. Schematic diagram of new general model
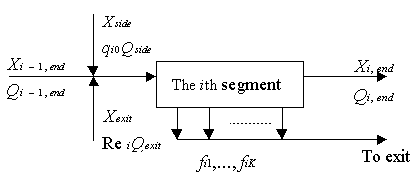
Figure 2. Schematic diagram of ith discretized model
Above is just an ideal situation and results
attained from it can not be directly realized. Then one possible discretized reactor
network model can be is derived, as Figure 2 shows. Theoretically speaking, the
discretized model can infinitely approach the primal model when segment number goes to
infinite.
1.2 Optimization problem developed
Based on above general model, an optimization model
P1 to solve reactor network synthesis problem is built. The objectives are generally the
function of exit concentrations and mean residence time. The Constraints consist of
material balances and logical constraints on distribution parameters. In P1, equation (1)
is differential mass balance expression. Equation (2) is initial feed condition. Equation
(3) is exit concentration expression. Equation (4) and (5) is normalization of f(t)
and q(t). Equation (6) is expression for mean residence time.
P1
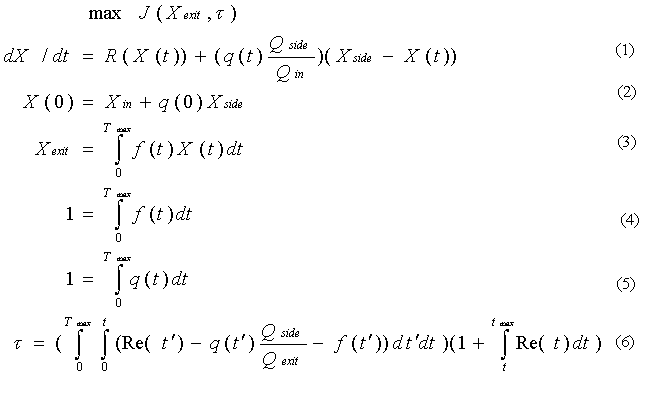
Orthogonal collocation on finite element method
(Cuthrell and Biegler, 1987) is applied to ensure the accuracy of the optimization
calculation that includes differential equations. The discretized model is a NLP
and can be directly solved by GAMS (Brooke and Kendrick, 1988). The resulted model
is P2.
P2
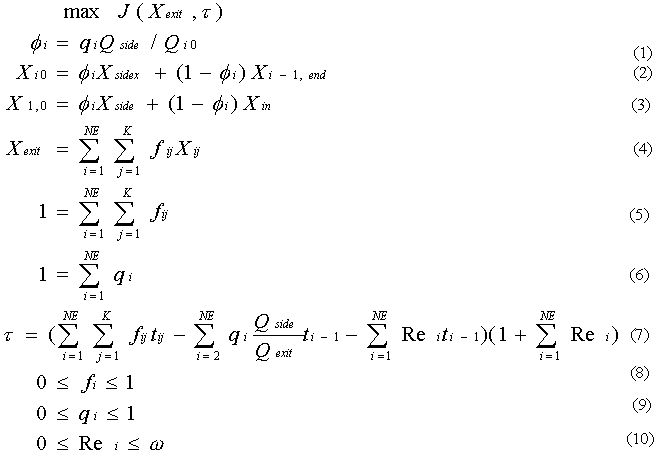
In P2, Equations (1) to (3) are expression
for concentrations at knots. Equation (4) is expression for temporary mixed concentration.
Equation (5) is the exit concentration expression. Equation (6) to (7) is normalization
expression Equation (8) is expression for mean residence time. Inequality constraints (9)
to (10) are constraints on distribution parameters.
2. MULTIOBJECTIVE OPTIMIZATION FORMULATION
In this paper, multi-objective optimization technique is used to solve the
waste minimization targeted reactor network design with maximum productivity as the
primary design objective and waste minimization as secondary objective. This is especially
important when the two objectives contradict and neither of them can be overlooked and
some trade-off between them should be considered. e constraint technique is applied to consider waste
minimization at the source to transform multi-objective problem into single objective
optimization problem. It can also be used to locate the convex and nonconvex regions of
the noninferior set. The multiobjective optimization problem P3 is formulated as P3.
P3
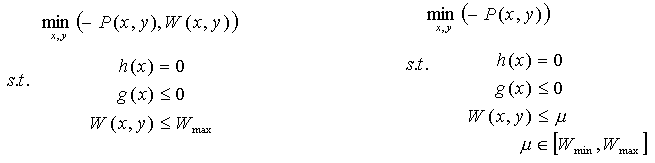
Where x is the set of network parameters, y is the
set of reaction network variables, m is a fixed parameter and h(x) and g(x) are the
constraints involved in optimization model P2. Above optimization model can be directly
applied to waste minimization design simply by choice of suitable objective function. The
upper and lower waste level WminWmax and Wmin are two limiting points attained separately by singly minimizing
waste amount and maximizing the product. When different upper lever of the waste is
assumed within the two waste limits, the correspondent productivity of P will be found.
Then we can map a noninferior curve by varying the waste level within its upper and lower
limits.
3. EXAMPLE PROBLEMS
3.1 Williams-Otto reaction system
Reactions and reaction rate expression for A, B, C, P, E and G is given as:
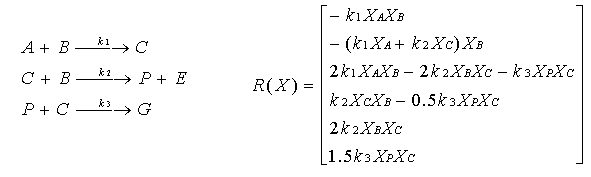
Reaction kinetic constants for k1, k2, k3
(h-1)are 6.1074, 15.0034 and 9.9851 respectively. Williams-Otto example is a
typical process integration problem and is largely used in reactor network synthesis
(Williams and Otto, 1968). Usually the reactor is simply recognized as a CSTR. A and B is
raw materials. P is the desired product. E is byproduct. C is intermediate product. G is
the waste. The upper and lower level of waste G is given in table 1. Figure 3 shows its
noninferior curve by choosing several points to calculate. It can be seen that the
noninferior curve has approximately two different segments, which is partly caused by the
strong interaction between side feeding and side recycling.
Table 1. Two limiting
points of the noninferior curve
|
OBJ Pmax (G) |
Network (t[hr]) |
|
OBJ Gmin (P) |
Network (t[hr]) |
Point
1 |
0.2432 (0.0895) |
DRDSR (0.03228) |
Point
2 |
0.00026 (0.0689) |
DRDSR (0.00548) |
This example has
illuminated the great influence of side feeding and recycling on waste generation. It can
be seen from calculation results that waste minimization and yield maximization objectives
are conflictive and some balance should be achieved. In the earlier paper where the effect
of side feeding and recycling on the yield of P and G is analyzed and conclusion was
reached that side feeding can greatly increase P and decrease G within some extent.
Further we can attain a noninferior curve that can meet the different need on the yield of
product P and waste G disposal regulation.
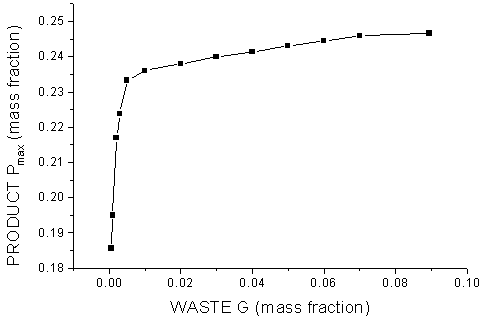
Figure 3. Noninferior curve for Williams-Otto
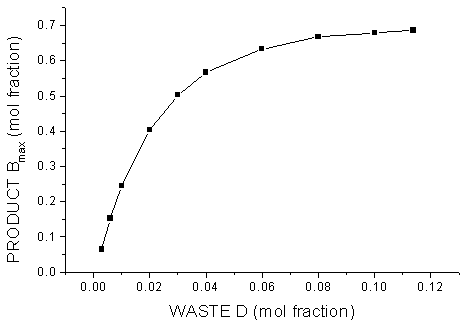
Figure 4. Noninferior curve for Van-de-Vusse
3.2 Van-de-Vusse Reaction system
Reaction equations and reaction
rate expression for A, B and C is given as:
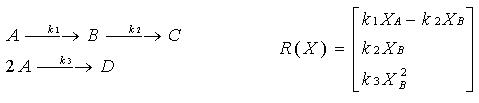
B is product and D is assumed to be waste
because it has great impact on the conversion of A to B as important reaction. Following
the same procedure with the maximization of B we can attain the noninferior curve as
figure 4 shows. From the results it can be seen that when the D upper level decreases, the
global recycling ratio increase accordingly. Unlike the complex situation in example 1,
the change of noninferior curve is quite smooth.
4. CONCLUSION
From the results, it can be concluded that since the side feeding,
recycling and bypassing strategy can give a comprehensive reflection of optimal
characteristic of the reactor network and the source reduction measure within the reactor
network, it can be effectively applied into waste minimization targeted design. The waste
minimization objective is stressed especially in this paper, which shows in two aspects:
outer multi-objective optimization to minimize waste level and inner source reduction
realization by two distribution side streams of side feed, recycling. So they can
effectively reduce the waste and provide good start point to realize source reduction.
Then multiobjective optimization is used to realize source reduction and yield
maximization at the same time to promote the profitability and environmental acceptability
of reactor network. In a conclusion, the three-distribution-parameters general model can
provide an effective tool to realize source reduction design to clean process.
NOMENCLATURE
i = index of finite element
j = index of collocation point
in = index of direct feeding
exit = index of exit of reaction network
side = index of side feeding
end = index of exit of each segment
f = residence time distribution parameter
k = reaction kinetics constant [hr-1]
q = side feeding distribution parameter
Q = mass flow rate [mol/(L sec)]
R = reaction rate [mol/sec]
Re = recycle parameter
t = reaction time [sec]
X = mol or mass fraction of species
P = productivity objective
W = waste objective
u = assumed upper lever of waste amount
Wmax
= maximum extent of waste
Wmin=
minimum extent of waste
a = segment
length [sec]
t = mean residence
time [sec]
f= ratio of side
feed flow to the bulk flow
w= upper limit
on recycling ratios
NE = number of finite elements
K = number of collocation points in each
element
Tmax
= maximum length of space time [sec]
REFERENCES
[1] Brooke A, Kendrick D, Meeraus A. GAMS: A User's Guide. Redwood City. CA: Science
Press, 1988.
[2] Cuthrell J E, Biegler L T. AIChE J, 1987, 33 (8): 1257.
[3] Lakshmanan A, Biegler L T. Pollution Prevention via Process and Product Modifications,
AIChE Symposium series, 94, 99 (303): 128.
[4] Schweiger C A, Floudas C A. Industrial & Engineering Chemistry Research, 1999, 38
(3): 744.
[5] Hua K L, Li Y R, Hu S Y et al. Computers and
Chemical Engineering, 2000, 24 (2): 217.
|