Polyoxometalate-based
organic-inorganic hybrid materials
Hu
Changwen, Wang Yonghui, Li Yangguang, Wang Enbo
(Institute of Polyoxometalate Chemistry, Faculty of Chemistry, Northeast Normal
University, Changchun, 130024, China)
Received Feb. 15, 2001; Supported by the
National Natural Science Foundation of China (20071007) and the Foundation for University
Key Teacher by the Ministry of Education of China.
Abstract The present paper summarizes and
reviews the current developments in the area of polyoxometalate (POM)-based
organic-inorganic hybrid materials including POM-based organic- or organometallic-derived
hybrid materials, charge transfer (CT) salts and organic-inorganic composite films.
POM-based organic- or organometallic-derived hybrids are reviewed in terms of four
subclasses, namely (i) ligands as charge-compensating cations, (ii) ligands bonded
directly to the POM framework, (iii) ligands bonded to the heterometal site and (iv)
organometallic ligands. Moreover, POM-based CT hybrid materials described here contain
different organic p-electron
donors such as substituted amides, aromatic amines, or electron-rich tetrathiafulvalenes
(TTF), bis(ethylenedithio)tetrathiafulvalene (BEDT-TTF or ET) as well as
decamethylferrocene (FeCp*2). Further, it is concluded that POM-based
organic-inorganic composite films can be prepared by four main strategies, namely doping
POMs in electrically conductive polymers, Langmuir-Blodgett technique, layer-by-layer
self-assembly method, and electrochemical growth method. Finally, this review also
provides insight into the rational synthesis and potential applications of these hybrid
materials.
Keywords polyoxometalate, organic-inorganic hybrid materials, structure, charge
transfer salts, composite films
1. INTRODUCTION
Organic-inorganic hybrid materials are of intensive interest in the field of contemporary
materials chemistry as these materials can exhibit synergetic properties such as
electrical, magnetic and optical properties[1].
Polyoxometalates (POMs) have been found wide applications in many
fields such as catalysis, medicine, magnetic properties, materials and surface chemistry,
and photo- and electrochromism owing to the so-called "value-adding
properties"[2]. The number of publications and
patents pertaining to POMs continues to grow. The scientific communities of the Peoples
Republic of China are becoming the important contributors of the total number of worldwide
publications on POMs. Figure 1 shows the countries where the most research activity exists
based on publications, in which the number of publications from China occupies the third
position (Russia the first and USA the second)[2].
It is noteworthy that POMs are receiving
increasing attention in the domain of materials science ascribing to their chemical,
structural and electronic versatility. A focus in this respect is the exploration of
organic-inorganic hybrid materials combining POMs and organic molecules and possessing a
variety of interesting conducting, magnetic, electronic and optical properties. So far,
tremendous advances have been made in this rapidly growing field[2].
The present review, covering the years 1995 to 2000, summarizes and
reviews the latest developments in the area of POM-based organic-inorganic hybrid
materials. The following section summarizes the efforts in POM-based organic- or
organometallic-derived hybrid materials. Section 3 presents the development of POM-based
charge transfer (CT) salts. Section 4 discusses progress in POM-based organic-inorganic
composite films. A summary and an outlook concerning the future of POM-based
organic-inorganic hybrid materials are presented in Section 5. In addition, only
structurally typical and representative POM-based hybrids are exemplified in this review
owing to the length limitation of this paper.
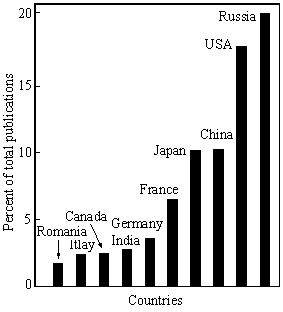
Fig. 1 The 10 countries with the largest number of POM
publications. Source: Chemical Abstracts ( till 1998)[2].
2. POM-BASED ORGANIC-INORGANIC HYBRID
MATERIALS CONTAINING ORGANIC OR ORGANOMETALLIC MOIETIES
Up till now, several synthetic methods have been adopted to prepare POM-based hybrid
materials containing organic or organometallic moieties, including routine processes[3-4],
hydrothermal reactions[5-10] and low-thermal solid phase synthesis[11].
Low-thermal solid phase synthesis, which is a novel synthetic strategy and involves the
direct reactions of POMs and organic molecules under grinding in an agate motar at room
temperature, has been applied to produce POM-based hybrids[11].
For clarity, the POM-based hybrids containing organic ligands reviewed
in the present paper are divided into three subclasses according to the role of organic
ligands: (1) charge-compensating cations, (2) ligands bonded directly to the POM skeletal
framework, and (3) ligands bonded to the heterometal site[5] (see Table 1).
Further, the hybrids containing organometallic moieties are listed as a fourth subclass
all alone (see Table 2).
Table 1 POM-based hybrids with organic
ligands
POM-based hybrid materials |
Structure |
Ref. |
Organic ligands as charge-compensating cations |
(C6H14N2)V6O14·H2O |
2D
layers |
[6] |
[N(CH3)4]5V18O46
|
2D
layers |
[7] |
(C6H18N2)[Mo4O13] |
1D
chains |
[8] |
[4,4'-H2bpy][Mo7O22]·H2O |
2D |
[9] |
[C12H25N(CH3)3]6(H2W12O40)·xH2O |
Keggin-type |
[10] |
[(n-C4H9)4N]6[FeIII4(H2O)2(PW9O34)2] |
sandwich-type |
[4] |
[(CH3)3(C6H5)N]4[(SiO4)W10MnIII2O36H6]·2CH3CN·H2O |
g-Keggin type |
[3] |
Organic molecules as ligands to the POM frameworks |
Nitrogen-containing ligands |
[MoO3(4,4'-bpy)0.5]
(MOXI-8) |
2D
layers |
[12] |
[HxMoO3(4,4'-bpy)0.5]
(MOXI-9) |
2D
layers |
[12] |
[Bu4N]2[Mo6O18(NR)]
(R=n-butyl, cyclohexyl, 2,6-(i-pr)2C6H3) |
molecular
cluster |
[13] |
[Bu4N]2[Mo6O19-n(NR)n]
(R=Ar, n = 1-6) |
molecular
cluster |
[13]
[14] |
Organosilyl ligands |
(n-Bu4N)3[(g-PW10O36)(t-BuSiOH)2] |
two
t-BuSiOH groups are linked to POM framework |
[15] |
Organophosphorous ligands |
[H2N(CH2CH2)2NH2][(VO){O3P(CH2)PO3}] |
1D
infinite puckered chains |
[16] |
[H3N(CH2)2NH3][(VO){O3P(CH2)2PO3}] |
2D
layers |
[16] |
[H3N(CH2)2NH3][(VO4)(OH)2(H2O)2{O3P(CH2)3PO3}2]·4H2O |
pillared
2D or 3D framework |
[16] |
(Et4N)H[g-SiW10O36(RPO)2]4-
(R=H, Et, n-Bu, t-Bu, C2H4COOH, Ph) |
g-Keggin type |
[17] |
Containing organic-ligated heterometals |
[M(4,
4'-bpy)(VO2)2(HPO4)4] (M=Co, Ni; bpy =
bipyridine) |
3D
containing helical chains |
[18] |
[Cu2(4,4'-bipy)2{Mo2O7}]n |
1D
chains |
[19] |
[H2en]2[{Cu(en)(OH2)}Mo5P2O23]·4H2O |
1D
spiral-shaped chains |
[20] |
[Cu(tpyor)Cu2Mo3O11] |
3D
microporous framework |
[21] |
[{Fe(tpyor)}3Fe(Mo6O19)2]·xH2O
tpyor=tetrapyridylporphyrin |
3D
microporous framework |
[21] |
[{Na(dibenzo-18-crown-6)}3{PMo12O40}]·5H2O |
molecular cluster |
[11] |
(Bu4N)3H2[g -SiO4W10O32(OH)Cr2(OOCCH3)2(OH2)2]·3H2O |
g-Keggin type |
[22] |
K6[Cu(Ala)2(H2O)2]2[Cu4(H2O)2(AsW9O34)2]·17H2O |
two Cu
complexes are supported on a sandwich-type POM |
# |
# Wang Y H, Hu C W, Peng J Wang E B,
Xu Y. Synthesis, characterization and X-ray crystal structure of a novel
polyoxotungstoarsenate: K6[{Cu(b-Ala)2(H2O)2}2{Cu4(H2O)2(AsW9O34)2}]·17H2O, J. Mol. Struc., 2001,
in press
2.1 Organic ligands as charge-compensating
cations in POM-based hybrids
Organic molecules such as organoamines have been extensively used as charge-compensating
cations in the synthesis of POM-based hybrids and a large number of such hybrids have been
prepared during these years (Table 1), as successfully exemplified by
polyoxovanadate-based hybrids. One typical structural feature of this kind of hybrids is
the insertion of organic molecules into the two-dimensional (2D) layered host framework of
polyoxovanadates[6-7]. A recent example is (C6H14N2)V6O14·H2O, which contains a new arrangement of edge-shared VO5
square pyramids that are corner-shared with VO4 tetrahedra to form highly
puckered layers, between which the 1,4-diazabicyclo[2.2.2]octane cations are sandwiched
(Fig.2)[6]. Another example is [N(CH3)4]5[V18O46][7].
The lattice is self-assembled from two distinct {V9O23} building blocks: One building block is neutral, while the other
carries localized electrons and is negatively charged. The organic cations reside between
the layers. During the formation of this hybrid, the organic cations act not only as a
templating agent but also as a reducing agent, and complicate the dissolution and
nucleation processes as well[7].
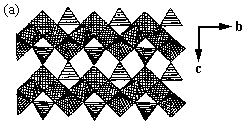 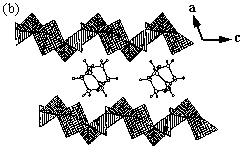
Fig. 2 (a) Portion of the
crystal structure of (C6H14N2)V6O14·H2O (along [100] that depicts four square-pyramidal VO5
units linked to form a single layer: Note the pairs of edge-sharing square pyramids that
have the same relative orientation; (b) the structure shown along [010].
The structure of a
one-dimensional (1D) molybdenum-containing organic-inorganic hybrid materials, (H3N(CH2)4NH3)[Mo4O13],
is interesting[8]. Its structure consists of bundles of parallel molybdenum
oxide chains interspersed with organic cations that play the role of intercalating agent
in the host structure.
Although a lot of vanadium- or molybdenum- containing organic-inorganic
hybrids have been prepared hydrothermally, tungsten-containing hybrids produced by
hydrothermal method were scarcely documented[10], most of which were
synthesized routinely, such as [(n-C4H9)4N]6[FeIII4(H2O)2(PW9O34)2][4]
and [(CH2)3C6H5N]4[(SiO4)W10Mn2O36H6]·CH3CN·H2O[3]
(see Table 1). The organic agents, for example, the tetra-n-butylammonium (TBA) cations,
usually act as precipitating agents or phase-transferring agents in the synthesis of these
hybrids.
2.2 Organic molecules as ligands to the POM frameworks
The direct bonding of organic ligands to POMs has greatly enhanced the structural
diversity of POM-based hybrid materials (Table 1).
Recently, Zubieta et al. reported three novel hybrids, [H2N(CH2CH2)2NH2][(VO){O3P(CH2)PO3}], [H3N(CH2)2NH3][(VO){O3P(CH2)2PO3}] and [H3N(CH2)2NH3][(VO4)(OH)2(H2O)2{O3P(CH2)3PO3}2]·4H2O, possessing
one-, two- and pillared two- or three-dimensional phase respectively[16]. They
have shown that the introduction of appropriate organic templates and modification of the
length of the organic buttress of the diphosphonato ligand can allow the engineering in
materials with different dimensions, specific interlayer spacings and hence tailored
microporosities.
In addition to being used as a cationic component (see Section 2.1),
the organoamines also act as ligands[12-14]. By raising the pH value of the
reaction medium to prevent protonation of the pyridyl nitrogens, [MoO3(4,4'-bpy)0.5]
(MOXI-8) and [HxMoO3(4,4'-bpy)0.5] (MOXI-9) were prepared
by Zubieta et al.[12]. The structure of MOX1-8 (Fig.3) contains layers of
corner-sharing {MoO5} square pyramids linked through
4,4'-bipyridyl groups into a three-dimensional (3D) covalently bonded framework, in which
alternating inorganic metal oxide layers and aromatic organic layers exist. The structure
of MOX1-9 is similar to that of MOX1-8, except that the octahedra adopt a more regular 5+1
type coordination, rather than the 2+2+2 type observed for MOX1-8[12].
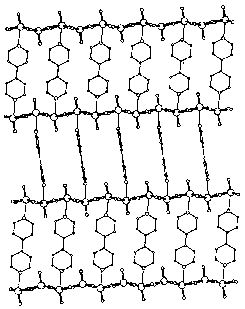
Fig. 3 View of the layer structure of [MoO3(4,4'-bpy)0.5]
(MOXI-8) parallel to the crystallographic a axis.
In routine organic solvent
synthesis, organoimide hexamolybdates constitute a new class of functionalized POMs, which
permit a broad range of appended groups to be incorporated through variations in the imide
substituent[13,14]. Recently, Maatta et al. reported multifunctionalized
organoimido derivatives of [Mo6O19]2-, in which a variety
of organoimido ligands are introduced at terminal oxo sites in the hexamolybdate cluster[13,14].
Moreover, polyvacant heteropolytungstates with organic groups anchored
to their backbones are another sort of interesting organic-inorganic hybrid materials and
have been receiving increasing attention during the past few decades[15,17].
Thouvenot et al. reported the [g-SiW10O36(RPO)2]4-[17] and
[g-PW10O36(t-BuSiOH)2][3-15].
The former is built up from a [g-SiW10O36]8- unit and two
phenylphosphonate (PhPO2+) groups which are linked to two oxygen atoms of two
edge-sharing WO6 octahedra. The structure of the latter is similar to that of
the former.
2.3 POM-based hybrids containing organic-ligated heterometals
The chemistry of POM-based hybrids is significantly enriched by the introduction of
heterometal coordination complexes into the hybrid systems (Table 1), either to provide
charge compensation or as part of the inorganic POM framework itself[5].
We have hydrothermally synthesized a novel POM-based hybrid containing
transition metal coordination complexes, [Cu2(4,4'-bipy)2{Mo2O7}]n
(bipy=bipyridyl)[19]. This hybrid exhibits a 1D chain structure consisting of
[Mo2O7]2- units and [Cu(4,4'-bipy)]nn+
chains (Fig.4). The [Mo2O7]2- units are covalently
bridged to two [Cu(4,4'-bipy)]nn+ chains, forming a hybrid with a
bridged double-chain structure.
Another example is represented by [M(4,4'-bpy)2(VO2)2(HPO4)4]
(M=Co or Ni, bpy=bipyridine), possessing a novel 3D structure constructed from two
subunits, namely [(VO2)(HPO4)]8 helical chains and
[M(4,4'-bpy)2]2+ fragments (Fig.5)[18]. Another
spiral-shaped chain [{Cu(en)(OH2)}Mo5P2O23]4-,
consisting of [Mo5P2O23]6- clusters connected
by {Cu(en)(OH2)O2}2+ square
pyramids, was reported two years ago[20]. Both helical hybrids containing
organoamine ligands, heterometals and especially PO4 units are reminiscent of
DNA structures, and maybe provide a potential route for the rational synthesis of
inorganic-organic mimetic systems[23].
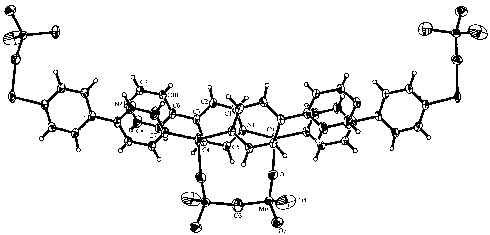
Fig. 4 A view of [Cu2(4,4'-bipy)2{Mo2O7}]n
showing 50% probability displacement ellipsoids and the atom-numbering scheme. H atoms
have been omitted for clarity.
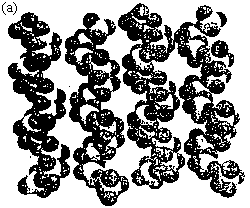 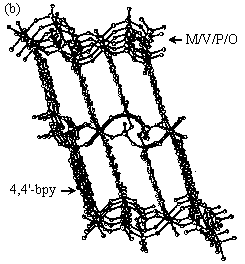
Fig.5 (a) View of the space-filling plot of
the left -helical and right-helical V-P-O chains. (b) View of the structure along the
(101) lane, showing the channels.
Zubieta and co-workers prepared
two novel hybrid materials: [Cu(tpyor)Cu2Mo3O11] and
[{Fe(tpyor)}3Fe(Mo6O19)2]·xH2O
(tpyor=tetrapyridylporphyrin) by hydrothermal reactions[21]. The structure of
the former could be described as neutral [Cu(tpypor)] network linked to bimetallic {Cu2Mo3O11} oxide chains, while the latter was constructed from a 3D cationic
framework [Fe4(tpyor)3]n4n+ and entrained {Mo6O19}2- cluster anions. The two structures illustrated that
molybdenum oxide structures might be readily modified by the porphyrin building blocks and
appropriate selection of porphyrin ligated metal centers, which can be considered as a
"ship in bottle" approach to modification of oxide microstructures[21].
It is noteworthy that nearly all the hybrids listed in Table 1 have
been made by hydrothermal technique, except (Bu4N)3H2[g-SiO4W10O32(OH)Cr2(OOCCH3)2(OH2)2]·3H2O[22], K6[Cu(b-Ala)2(H2O)2]2[Cu4(H2O)2(AsW9O34)2]·17H2O# and [{Na(dibenzo-18-crown-6)}3{PMo12O40}]·5H2O [11]. The first two hybrids were prepared
by routine method. In the structure of the first hybrid, the Cr2(OOCCH3)22+
units occupy the vacant sites of the g-SiW10O40 units. Recently, we have prepared a
novel POM-based hybrid compound, K6[{Cu(b-Ala)2(H2O)2}2{Cu4(H2O)2(AsW9O34)2}]·17H2O (b-Ala=b-alanine), which can be described as two [Cu(b-Ala)2(H2O)2]2+
fragments coordinated with the sandwich-type [Cu4(H2O)2(AsW9O34)2]10-
unit through bridging oxo groups (Fig.6).
More recently, using low-thermal solid-phase method, You et al.
obtained a yellow crystal [{Na(dibenzo-18-crown-6)}3{PMo12O40}]·5H2O through grinding the
mixture of the crown ether and H3PMo12O40 (Fig.7)[11].
Its structure consists of three {Na(dibenzo-18-crown-6)} units coordinated with a triad of
addenda metals of the polyoxoanion through the terminal oxo groups. To our knowledge, this
is the first crystal whose Keggin-type polyoxoanion is connected with crown ether.
|
|
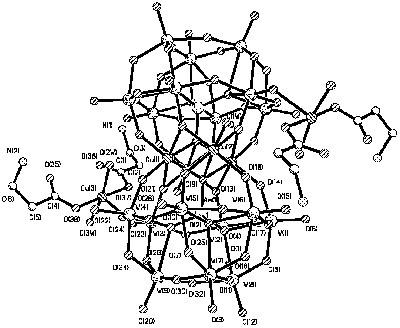 |
Fig. 6 View of the structure
of [{Cu(b-Ala)2(H2O)2}2{Cu4(H2O)2(AsW9O34)2}]6+ |
|
|
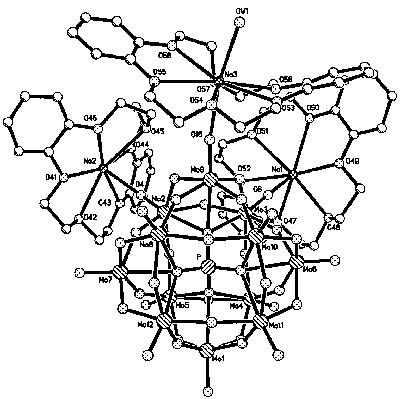 |
Fig. 7 View of the crystal
structure of [{Na(dibenzo-18-crown-6)}3{PMo12O40}]·5H2O |
2.4 POM-based hybrids containing
organometallic moieties
The chemistry of POM-based hybrids containing organometallic moieties is an area of
growing interest[24-29]. Generally these hybrids can be classified into three
types based on their structures: (i) organometallics supported on a POM, (ii)
organometallics incorporated into a POM and (iii) integrated cubane-type clusters[2].
As to the difference between (i) and (ii), Finke has given a clear explanation[30].
POM-supported organometallic hybrids mean species that the organometallic moiety is firmly
attached to a k3-O
site of surface oxygens of the support POM, while in POM-incorporated organometallics, the
organometallic moiety is incorporated into a vacancy in the POM framework by four
approximately square-planar oxo ligands.
Quite recently, a series of new POM-based organometallic hybrids have
been synthesized (Table 2). Two POM-incorporated hybrids, [PW11O39RhCH2COOH]5-
and [SiW11O39RhCH2COOH]6-, were obtained by
the hydrothermal method[27].
Owing to the fact that surface activation of POMs can be achieved by
replacing the metal (Mo(VI) or W(VI)) centers by one or more lower valent metals, a number
of substituted-type POM-supported organometallic hybrids have been synthesized. For
example, reaction of [PW11O39NbO]4- with Cp2Zr(Otf)2THF
(Cp=C5H5-, Otf=O3SCF3-) in acetonitrile
yielded the first POM-supported biscyclopentadienyl complex of Group 4, [(PW11O39NbO)2ZrCp2]6-
[24].
A series of integrated cubane-type clusters have been developed as
potential models for inorganic solid surfaces, e.g. [{Ru(h6-p-MeC6H4Pri)}4Mo4O16]
[29]. In solid state, the hybrid displays the so-called windmill-like structure,
i.e. it consists of a [Mo4O16]8- cubic core capped by
four {Ru(h6-p-MeC6H4Pri)}2+
groups each bound to a triply bridging and two terminal oxo ligands.
Table 2 POM-based hybrids with
organometallic ligands
POM-based hybrids |
Structure |
Ref. |
[(PW11O39NbO)2ZrCp2]6- |
POM-supported |
[24] |
[(n-C4H9)4N]5Na3[(1,5-COD)RhâP2W15Nb3O62] |
POM-supported |
[25] |
2CH3CN
(n-Bu4N)2[{Ir(1,5-COD)}6W4O16]·2CH3CN |
POM-supported |
[28] |
[(PhSn)3Na3(a-SbW9O33)2]6-,
[{(PhSn)2O}2H(a-AsW9O33)2]9- |
POM-incorporated |
[26] |
[XW11O39RhCH2COOH]5,6-
(X = P, Si) |
POM-incorporated
|
[27] |
[{Ru(h6-p-MeC6H4Pri)}4]Mo4O16 |
cubane-type |
[29] |
3. POM-BASED CHARGE TRANSFER (CT) SALTS
A recent development of POMs in materials science deals with the use of soluble
metal-oxide clusters as inorganic electron-accepting moieties in new organic-inorganic CT
hybrid materials based on organic p-electron donors such as substituted amides[31],
aromatic amines[40], or electron-rich substrates such as tetrathiafulvalenes
(TTF)[2,32], bis-(ethylenedithio)tetrathiafulvalene (BEDT-TTF or ET)[33-36]
or decamethylferrocene (FeCp*2) [39]. The combination of these
organic donors with POMs of various nuclearities, shapes, and charges has resulted in new
types of magnetic, insulating, semiconducting, or even metallic CT salts. Table 3 gives a
list of this kind of hybrid materials recently synthesized.
Table 3 Recently synthesized POM-based CT
salts
POM-based CT salts |
Property |
Ref. |
H4SiW12O40·4HMPA·2H2O
(HMPA=Hexamethylphosphoramide) |
I2w =0.7 I2w KDP and c(3)=2.63×10-11
esu |
[31] |
[TTF]3[S2Mo5O23]2(NMe4)6·2MeCN (NMe4+=tetramethylammonium) |
|
[2] |
[bis(TTF)]2[SiMo12O40]·10H2O
(bis(TTF)=2,6-[4,5-bis(n-butylsulfanyl)-1,3-dithiol-2-yldene]-4,8-bis(6-iodo-n-hexyloxy)-1,3,5,7-tetrathia-s-indacene) |
semiconductor
(sRT=1.3×10-4
S cm-1) |
[32] |
[ET]8[SiMo12O40]·10H2O |
semiconductor
(sRT=1.5×10-3
S cm-1) |
[33] |
a2-ET8[XZ(H2O)M11O39]
(XZM11=SiFeMo11, SiCrW11, PZnW11, PCoW11,
PCuW11 and PNiW11),
a3-ET8n[PMnM11O39]n
(M=W and Mo) |
cmT=1.5~0.2 emu·K·mol-1 from room temperature (RT) to 2 K (PZnW11) |
[34] |
[ET]11[P2W18O62]·3H2O |
a
metallic-like behavior (sRT=5
S cm-1 to 5.5 S cm-1 at 230 K) and semiconductor below 230 K |
[35] |
[ET]6(H)4[M4(H2O)2(PW9O34)2] |
insulators
and ferromagnetic clusters |
[36] |
(BEST)3H[PMo12O40]·CH3CN·CH2Cl2
(BEST=
Bis(ethylenediseleno)tetrathiafulvalene) |
strong
antiferro-magnetism |
[37] |
[BET]2[M6O19]
(M=W, Mo) |
insulators
(sRT=3.2×10-6
S cm-1) |
[38] |
[Fe(C5Me5)2]3[Cr(OH)6Mo6O18]·20H2O [FeCr(1)],
Na[Fe(C5H5)2]2[Cr(OH)6Mo6O18]·3H2O [FeCr(2)],
[Fe(C5Me5)2]4[HPCu(H2O)W11O39]·6CH3CN (FeCu) |
weak
antiferromagnetic properties
cmT=
4.7~4.3 emu·K·mol-1 from
RT to 2 K [FeCr(1)] |
[39] |
Coronado and
co-workers have prepared the first well-characterized ET-type CT salt of the Dawson-Wells
polyanion [P2W18O62]6-, (ET)11[P2W18O62]·3H2O, consisting of alternating layers of anions and ET
molecules in the ac plane[35]. The Dawson-Wells polyanion has induced a b packing in the organic ET component,
formed by an exotic zigzag chain arrangement of six independent ET molecules. This hybrid
has very interesting electrical properties. It exhibits a metallic-like behavior
characterized by increased conductivity from ~5 S cm-1 at room temperature to
5.5 S cm-1 at 230 K. Below this temperature the hybrid becomes a semiconductor
with a very low activation energy value (Fig.8). This result illustrates how a change in
the packing of the organic molecules (from a in the Keggin phases to b in the Dawson-Wells derivative) can give rise to hybrid molecular
materials with higher conductivities despite the large size of the Dawson-Wells anion. The
magnetic properties of this compound indicates the diamagnetic nature of this compound.
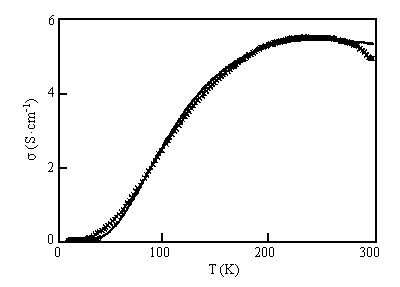
Fig. 8 Thermal variation of the dc electrical conductivity of [ET]11[P2W18O62]·3H2O in the ac plane. The solid line is the fit to
a semiconductor model with a very small activation energy (Ea=27 meV) in which the
effect of the electron mobility on the conductivity has been taken into account.
We have prepared a CT salt
[C42H60Cl2O2S12]2[SiMo12O40]
based on p-extended
bis(TTF) and a Keggin polyoxoanion[32]. In the crystal structure, organic
radical dications and silicamolybdate anions are alternatively arranged along a axis to
form a 1D conducting layer. The room-temperature d.c. conductivity determined by three
two-probe method is 10-4 S cm-1, suggesting that the compound is a
semiconductor. Subsequently, we reported another POM-based CT hybrid, [ET]8[SiMo12O40]·10H2O, consisting of 2D organic and inorganic sublattices
alternating along the unique axis [010] of the monoclinic unit cell. Its conductivity was
lower than those of other ET salts with Keggin anions due to the longer
chalcogen-chalcogen distances[33].
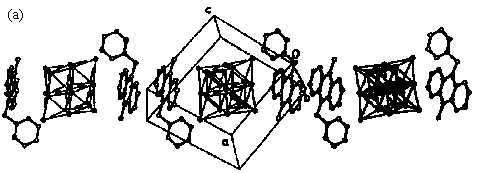
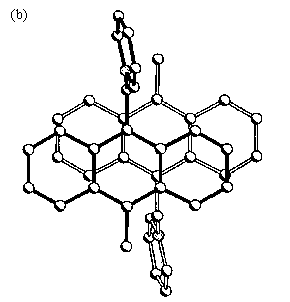
Fig. 9 (a) Infinite stacks of anthracene donor and polyoxometalate
acceptor with DAD/DAD/DAD sequence in the crystal structure of (Me-ANT-CH2-Py+)2[Mo6O19]2-
salt. (b) Overlap of "dimeric"
anthracene nuclei in the crystal structure of (Me-ANT-CH2-Py+)2[Mo6O19]2-
salt.
More recently, novel CT
hybrid materials have been prepared via cocrystallization of planar aromatic donors
(anthracenes and pyrenes) and spherical POM acceptors such as Lindqvist-type [M6O19]2-
and Keggin-type [SiM12O40]4- (M = Mo or W) through
attaching a cationic "anchor"
onto the arene which then clings to the POM anion by Coulombic forces[40]. A
unique arrangement of the arene donors and the hexamolybdate acceptors, namely a
DAD/DAD/DAD... sequence, is observed in the stacking (see Fig.9 (a)), where two
neighboring donor moieties are cofacially oriented with an interplanar distance of 0.346
nm and embedded between the spherical POM units. This allows a high degree of p-orbital overlap (see Fig.9 (b)) and
results in satisfaction of the 2:1 stoichiometry based on the ionic charges and
accommodation of the different shapes and sizes of donor and acceptor. Furthermore, an
interesting logical structure/property relationship is observed, that is, the shorter the
distance between the POM surface and the arene nucleus, the darker is the color of the CT
crystal and the faster is the decay of the laser-excited charge-transfer state (due to
back-electron transfer). Thus, these hybrid materials may be interesting candidates for
nonlinear optical and/or ferromagnetic properties.
Furthermore, Golhen et al. have reported several molecular assemblies
of POM-based ferrocenium hybrids, including ([Fe(C5Me5)2]3[Cr(OH)6Mo6O18]·20H2O [FeCr(1)], Na[Fe(C5H5)2]2[Cr(OH)6Mo6O18]·3H2O [FeCr(2)], and [Fe(C5Me5)2]4[HPCu(H2O)W11O39]·6CH3CN (FeCu)[39]. In the hybrid FeCr(1), the
Fe(C5Me5)2 units are perpendicular to each other and form
a centrosymmetrical group of three cations, which alternates with the planar [Cr(OH)6Mo6O18]3-
anion to form perpendicular mixed chains. In the hybrid FeCu, the decamethylferrocenium
units form a polyhedral structure that encapsulates the Keggin polyanion. The magnetic
properties indicate that the hybrids containing metallocenium radicals present weak
antiferromagnetic interactions obeying the Curie-Weiss law.
You et al. have reported a POM-based CT salt, a-H4SiW12O40·4HMPA·2H2O, composed of the
heteropolytungstate a-H4SiW12O40
and the organic substrate hexamethylphosphoramide (HMPA)[31]. The complex has
no photosensitivity under irradiation of sunlight, but under the near-UV light result in a
charge transfer by oxidation of the HMPA and the reduction of the POM. This hybrid shows a
certain second-order and third-order nonlinear optical response of I2w = 0.7I2w KDP and c(3) = 2.63 ×10-11
esu, respectively.
4. POM-BASED ORGANIC-INORGANIC COMPOSITE
FILMS
As aforementioned, POMs have been widely used in many fields such as conductivity,
magnetism, electrocatalysis, photochromism and molecular electronics[2].
However, practical applications of POMs in these areas rely largely on the successful
fabrication of POM-based organic-inorganic films. Recently, several main strategies have
been adopted to prepare lamellar POM-based organic-inorganic hybrids, including (i) doping
POMs in electrically conductive polymers[36,41]; (ii) Langmuir-Blodgett
technique[42-47]; (iii) layer-by-layer self-assembly method[49-53]
and (iv) electrochemical growth method[54-56].
4.1 Composite films formed by doping POMs in electrically conductive polymers
By embedding or incorporating POMs within conducting polymers such as polypyrrole,
polyaniline and polythiophene, a number of POM-based organic-inorganic composite films as
well as their bulk materials have been prepared in recent years, which can be used as
conducting electrodes or cation-insertion electrodes. A more commonly used incorporation
method is electrochemical oxidation of a monomer under a suitable oxidation potential by
using a POM as the electrolyte. Thus, the conductive polymer is deposited on the working
electrodes (usually carbon or graphite) doped with the polyoxoanions.
Gómez-Romero et al. have both chemically and electrochemically
synthesized a POM-based hybrid film (C4H3N)9(PMo12O40)
formed by polypyrrole and the phosphomolybdate anion [PMo12O40]3-
[41]. The 9:1 stoichiometry agrees with the expected value for a fully oxidized
polymer with the anion [PMo12O40]3- balancing the
charges. This hybrid maintains the good conductivity of the polymeric matrix and presents
the added electrochemical activity of the inorganic clusters. Its electrochemical cycling
is found to involve the insertion and de-insertion of cations during reduction and
oxidation process respectively, representing a key modification of the cycling mechanism
of polymeric electrodes. Thus, the present work establishes the function of these hybrid
materials as electrodes taking advantage of the reversible electrochemical activity of the
POM clusters.
Clemente-León and co-workers have electrochemically prepared the
organic/inorganic films consisting of the [Co4(H2O)2(PW9O34)2]10-
polyoxoanion incorporated in polypyrrole (~ 80 pyrrole units per cobalt cluster)[36].
The magnetic properties are very close to those observed in the K+ salt,
indicating that the structure of the ferromagnetic cluster is maintained in the film. The
electrical properties reveal a semiconducting behavior. This hybrid represents the first
hybrid film formed by a high spin cluster embedded in a polypyrrole polymer in
which the large magnetic moments localized on the POM coexist with a de-localized electron
framework. The authors have claimed that such a strategy is general and can be extended to
other kinds of magnetic POMs and conducting films.
4.2 Composite films formed by Langmuir-Blodgett deposition technique
Recently, Langmuir-Blodgett technique has been extended to create organic-inorganic LB
films containing well-organized layers of polyoxoanions[42-47]. As a matter of
fact, this method has been confirmed as a useful tool to improve the order and properties
of these new lamellar hybrid materials (see below).
Using the adsorption properties of Keggin heteropolyanions along a
positively charged monolayer of a lipid such as the dimethyldioctadecylammonium cation
(DODA), Clemente-León et al.[42-43] have constructed new organic/inorganic
films DODA/XW12O40 (X=2(H+), PV, SiIV,
BIII, CoII). The control of the Keggin polyanion amount in the
multilayers is made possible by mixing DODA with a negatively charged lipid, which
modifies the global electrical charge of the Langmuir film. The magnetic behavior of the
DODA/CoW12O40 LB films has been measured and found to follow the
Curie law down to about 20 K, as expected for an isolated CoII in a tetrahedral
environment. Below this temperature, the magnetic moment decreases because of the
zero-field splitting of the S = 3/2 CoII ion similar to the behavior observed
for the potassium salt of this anion. Thus it is demonstrated that the magnetic site of
the polyanion embedded in the LB film is not sensitive to the surrounding charge of the
DODA molecules.
The same group has extended this LB method to other POMs and prepared
three new LB films, that is DODA/K10[Co4(H2O)2(PW9O34)2],
DODA/K6[SiMn(H2O)W11O39] and DODA/K16[Co4(H2O)2(P2W15O56)2][44,45].
These POMs are well organized in monolayers within the LB films. The magnetic properties
of these films are measured and found to be very close to those obtained in the K+
salts.
Kurth et al. have fabricated the LB films of a surfactant-encapsulated
cluster (SEC), (DODA)20(NH4)[H3Mo57V6(NO)6O183(H2O)18][46].
The film thickness for the first layer is 2.6 nm and an average film thickness is 2.3 nm
for subsequent layers. These values are smaller than the diameter of the SEC, but packing
effects within the films can reduce the apparent thickness per layer. Deposition is
observed to be very reproducible and film growth essentially linear, that is to say, in
each dipping cycle equivalent amounts of SEC are transferred on the substrate (transfer
ratio 0.95). In addition, the resulting LB films are completely hydrophobic, which
demonstrates the efficient encapsulation of the hydrophilic cluster by the surfactant
molecules. Subsequently, the LB films of another SEC, (DODA)40(NH4)2[(H2O)n
Mo132O372(CH3COO)30(H2O)72],
was reported by the same group[47]. Since this SEC possesses a nanoporous
structure, its LB films would certainly represent a completely novel type of nanoreactors,
from which functional nanodevices may evolve.
The aforementioned contributions clearly indicate that LB technique can
be extended to all types of polyanions or charged clusters, giving rise to new lamellar
organized materials that have particular magnetic, optical, electrochromic, or
electrochemical properties. However, this still depends on the appropriate choice of both
POMs and lipid molecules.
4.3 Composite films formed by layer-by-layer self-assembly method
The layer-by-layer self-assembly method originally applied to prepare multilayer
assemblies of organic polymers[48] has recently been used to produce ordered
POM-based organic-inorganic films[49-53]. This technique involves the
sequential adsorption of oppositely charged species from dilute solution and is
particularly attractive because of its simplicity and effectiveness in creating multilayer
films on various substrates and allowing the easy control of the desired order and number
of layers at the nanometer level.
In 1996, Kuhn et al.[49] obtained the deposits of
multilayers of Os(bpy)32+-P2Mo18O626-
on different electrode surfaces by using this method. The composition of the multilayers
was found to depend on the identity of the electrode surface, the pH of the deposition
solution, and the electrochemical treatments applied during the deposition steps.
Later, Ichinose et al.[50] reported the self-assembled
ultrathin multilayers formed by (NH4)4[Mo8O26]
and poly(allylamine) hydrochloride (PAH) (Fig.10). In this work, adsorption of (NH4)4[Mo8O26]
on PAH surfaces was found to proceed without saturation and the thickness of the oxide
layer in the individual adsorption step increased with the adsorption time at a rate of
ca. 0.57 nm/min, due to the condensation of the adsorbed octamolybdate and the resulting
loss of negative charges. The thickness of the POM layer can be controlled in a range of
2~25 nm by the adsorption times employed, corresponding to 2~30 layers of the [Mo8O26]4-
unit. In addition, the formation of the multilayer films was found to critically depend on
the pH of the polymer solutions (pH~3).
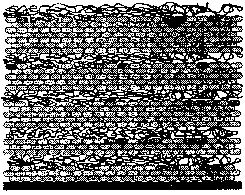
Fig. 10 Schematic illustration of a
molybdenum oxide/PAH multilayer. The first two oxide layers are composed of three layers
of [Mo8O26]4- units corresponding to the 4- and 7-min
adsorptions of (NH4)4[Mo8O26]. PAH layers have
a constant thickness of ca. 3 nm.
Moriguchi et al.[51]
prepared the ultrathin films self-assembled from poly (diallyldimethylammonium) chloride
(P) and sodium decatungstate (W) on various substrates (S) and also studied the
electrochromic properties of these films. The amount of W adsorbed on quartz in one
self-assembly step is ca. 2~3 times greater than that evaluated from the geometric area of
this molecule, suggesting that W10O324- penetrates into
and adsorbs onto an underlying P layer to form a P-W ion complex. In addition, the
concentration of W in S-(P/W)n films was almost constant irrespective of the
species of the substrates. The author also confirmed the stable electrochromic property of
the self-assembled S-(P/W)n/P films.
Also in 1998, Caruso et al.[52] produced ultrathin
multilayer films of a novel molybdenum (VI) POM cluster (NH4)21[H3Mo57V6(NO)6O183(H2O)18]
(Mo57) and PAH by this method. The Mo57 density in the film could be
readily controlled by varying the polyelectrolyte interlayer separation between each Mo57
layer, and the total film thickness could be controlled by altering the number of
adsorption cycles.
More recently, the same group[53] has reported another kind
of stable ultrathin composite films consisting of polyallylamine hydrochloride (PAH) and
the nanoporous isopolyoxomolybdate [(H2O)n Ì Mo132O372(CH3COO)30(H2O)72]42-
(Mo132) fabricated by the layer-by-layer self-assembly method. The deposition
of the Keplerate Mo132 was found to be highly reproducible and the average
effective film thickness for a Mo132/PAH layer pair was 2.2±0.2 nm. The
measured film thickness of a single Mo132 layer amounts to ~1 nm. The remaining
1.2-nm film thickness is, therefore, attributed to PAH.
On the whole, in contrast to LB technique, the layer-by-layer
self-assembly method appears more facile, versatile and promising in fabricating POM-based
organic-inorganic composite films with nanocontrol. However, it is crucial to understand
the factors controlling the film growth and precise thickness, such as the adsorption
time, the solution pH value and the polycations employed.
4.4 Composite films formed by electrochemical growth method
Dong et al. have developed a novel electrochemical growth method involving cyclic
potential sweeps (CPS) over a suitable potential range in modifier solutions to produce
POM-containing organic-inorganic films[54-56]. A successful example is
composite films based on SiMo11VO405- and a cationic
polymer of quaternized poly(4-vinylpyridine) partially complexed with osmium
bis(2,2'-bipyridine) chloride[54]. These films not only are uniform and
homogeneous, but also exhibit well-defined surface waves characteristic of the POMs' redox reactions. This electrochemical strategy has also been
successfully popularized by the same group to other structurally different POMs[55-56].
By comparing this electrochemical method with the immersion method, the
authors conclude that the electrochemical growth may provide at least two advantages in
favor of the multilayer growth[54]. First, a positive electric field is formed
in electrode interface by CPS in a positive potential window. It must accelerate and suit
the deposition of highly negatively charged polyanions. On the other hand, by
electrostatic repulsion, it avoids the drawback of overdeposition. Second, redox reactions
between heteropolyacids and heteropolyblues (HPAÛHPB) during CPS should be more adaptable to loading polyanions,
since the resulting HPBs are known as complex colloidal species, bearing much higher
negative charges.
In short, the electrochemical growth method is claimed to be more
advantageous than the immersion growth and suitable for anchoring nearly all kinds of
POMs, including those unable to be modified by the previous strategies[54].
5. SUMMARY AND OUTLOOK
In this review, we have presented the latest developments in the newly emerging area of
POM-based organic-inorganic hybrid materials. So far, the number of these hybrid materials
has been continuously and rapidly growing owing to their potential applications in
catalysis, medicine and materials science. However, the rational design and synthesis of
this kind of hybrid materials with desired structures and specific properties is still a
challenge to synthetic chemists. Furthermore, new synthetic routes and strategies need
development in order to enrich the chemistry of POM-based organic-inorganic hybrid
materials.
REFERENCES
[1] Kagan C R, Mitzi D B, Dimitrakopoulos C D. Science, 1999, 286: 945.
[2] Hill C L. Chem. Rev., 1998, 98 (1): 1.
[3] Zhang X Y, O'Connor C J, Jameson G B et al. Inorg. Chem.,
1996, 35 (1): 30.
[4] Zhang X, Chen Q, Duncan D C et al. Inorg. Chem., 1997, 36 (20): 4381.
[5] Hagrman P J, Hagrman D, Zubieta J. Angew. Chem. Int. Ed., 1999, 38 (18): 2638.
[6] Nazar L F, Koene B E, Britten J F. Chem. Mater., 1996, 8 (2): 327.
[7] Koene B E, Taylor N J, Nazar L F. Angew. Chem. Int. Ed., 1999, 38 (19): 2888.
[8] Xu Y, An L H, Koh L L. Chem. Mater., 1996, 8 (3): 814.
[9] Zapf P J, Haushalter R C, Zubieta J. Chem. Commun., 1997 (3): 321.
[10] Janauer G G, Dobley A, Guo J et al. Chem. Mater., 1996, 8 (8): 2096.
[11] You W, Wang E, Li Y et al. Inorg. Chem., 2001, in press.
[12] Hagrman P J, LaDuca R L, Koo H J et al. Inorg. Chem., 2000, 39 (19): 4311.
[13] Strong J B, Yap G P A, Ostrander R et al. J. Am. Chem. Soc., 2000, 122 (4): 639.
[14] Strong J B, Haggerty B S, Rheingold A L et al. Chem. Commun., 1997 (12): 1137.
[15] Mazeaud A, Dromzee Y, Thouvenot R. Inorg. Chem., 2000, 39 (21): 4735.
[16] Soghomonian V, Chen Q, Haushalter R C et al. Angew. Chem. Int. Ed. Engl., 1995, 34
(2): 223.
[17] Mayer C R, Herson P, Thouvenot R. Inorg. Chem., 1999, 38 (26): 6152.
[18] Shi Z, Feng S, Gao S et al. Angew. Chem. Int. Ed., 2000, 39 (13): 2325.
[19] You W S, Wang E B, Xu L et al. Acta Cryst., 2000, C56, 289.
[20] Lu J, Xu Y, Goh N , et al. Chem. Commun., 1998 (24): 2733.
[21] Hagrman D, Hagrman P J, Zubieta J. Angew. Chem. Int. Ed., 1999, 38 (21): 3165.
[22] Wassermann K, Lunk H-J, Palm R et al. Inorg. Chem., 1996, 35 (11): 3273.
[23] Soghomonian V, Chen Q, Haushalter R C et al. Science, 1993, 259, 1596
[24] Radkov E V, Young Jr. V G, Beer R H. J. Am. Chem. Soc., 1999, 121 (38): 8953.
[25] Aiken J D, Finke R G. Chem. Mater., 1999, 11 (4): 1035.
[26] Sazani G, Dickman M H, Pope M T. Inorg. Chem., 2000, 39 (5): 939.
[27] Wei X, Dickman M H, Pope M T. J. Am. Chem. Soc., 1998, 120 (39): 10254
[28] Hayashi Y, F Mü1ler, Lin Y et al. J. Am. Chem. Soc., 1997, 119 (47): 11401.
[29] Artero V, Proust A, Herson P et al. Chem. Commun., 2000 (10): 883.
[30] Finke R G, Rapko B, Domaille, P J. Organometallics, 1986, 5, 175.
[31] Niu J Y, You, X Z, Duan, C Y et al. Inorg. Chem., 1996, 35 (14): 4211.
[32] Peng J, Wang E, Zhou Y et al. J. Chem. Soc., Dalton Trans., 1998 (22): 3865.
[33] Peng J, Wang E, Xin M et al. Polyhedron, 1999, 18, 3147.
[34] Coronado E, Galán-Mascarós J R, Giménez-Saiz, C et al. J. Am. Chem. Soc., 1998,
120 (19): 4671.
[35] Coronado E, Gálan-Mascarós J R, Giménez-Saiz C et al. Adv. Mater., 1996, 8 (10):
801.
[36] Clemente-León M, Coronado E, Galán-Mascarós J R et al. J. Mater. Chem., 1998 (2):
309.
[37] Coronado E, Galán-Mascarós J R, Giménez-Saiz C et al. Inorg. Chem., 1998, 37 (9):
2183.
[38] Coronado E, Galán-Mascarós J R, Giménez-Saiz C et al. J. Mater. Chem., 1998 (2):
313.
[39] Golhen S, Ouahab L, Grandjean D et al. Inorg. Chem., 1998, 37 (7): 1499.
[40] Le Maguerés P, Hubig S M, Lindeman S V et al. J. Am. Chem. Soc., 2000, 122 (41):
10073.
[41] Gómez-Romero, P, Lira-Cantu, M. Adv. Mater., 1997, 9 (2): 144.
[42] Clemente-Leon M, Agricole B, Mingotaud C et al. Langmuir, 1997, 13 (8): 2340.
[43] Clemente-Leon M, Mingotaud C, Agricole B et al. Angew. Chem. Int. Ed. Engl., 1997, 36
(10): 1114.
[44] Clemente-Leon M, Mingotaud C, Gomez-Garcia C J et al. Thin Solid Films, 1998, 327 329, 439.
[45] Clemente-Leon M, Mingotaud C, Gomez-Garcia C J et al. Synth. Met., 1999, 103, 2263.
[46] Kurth D G, Lehmann P, Volkmer D et al. Chem. Eur. J., 2000, 6 (2): 385.
[47] Volkmer D, Du Chesne A, Kurth D G et al. J. Am. Chem. Soc., 2000, 122 (9): 1995.
[48] Decher G. Science, 1997, 277, 1232.
[49] Kuhn A, Anson F C. Langmuir, 1996, 12 (22): 5481.
[50] Moriguchi I, Fendler J H. Chem. Mater., 1998, 10 (8): 2205.
[51] Ichinose I, Tagawa H, Mizuki S et al. Langmuir, 1998, 14 (1): 187.
[52] Caruso F, Kurth D G, Volkmer D et al. Langmuir, 1998 (13): 14, 3462.
[53] Kurth D G, Volkmer D, Ruttorf M et al. Chem. Mater., 2000, 12 (10): 2829.
[54] Cheng L, Niu L, Gong J et al. Chem. Mater., 1999, 11 (6): 1465.
[55] Liu S, Tang Z, Shi Z et al. Langmuir, 1999, 15 (21): 7268.
[56] Tang Z, Liu S, Wang E et al. Langmuir, 2000, 16 (13): 5806.
|