Derivative hydride generation atomic
absorption spectrometric determination of tin at sub-mgL-1 in water samples
Sun
Hanwen, Liang Shuxuan, Ha Jing, Kang Weijun
(Key Laboratory of Analytical Science and Technology of Heibei Province, College of
Chemistry and Environmental Science, Hebei University, Baoding, 071002, China)
Received Dec. 6, 2002; Supported by the
Natural Science Foundation of Hebei Province for the project (No. 296024).
Abstract A
new method was described for the direct determination of tin at sub-mg·L-1 levels in environmental waters by
derivative hydride generation atomic absorption spectrometry with boracic acid as the
reaction medium. The signal model and fundamentals of the derivative hydride generation
atomic absorption spectrometry (DHGAAS) were described. The effects of the reaction
medium, KBH4 concentration and atomization temperature were investigated and
analytical conditions were optimized. The sensitivity for tin detection was increased 43
times better than those of conventional hydride generation atomic absorption spectrometry
(HGAAS). For a 2 mV·min-1 sensitivity range setting, the characteristic concentration for
tin was 0.004 mg·L-1,
and the detection limit (3s ) for tin was 0.021mg·L-1. The relative standard deviations of 7
replicate determinations of tin in water samples at 0.08-0.72mg·L-1 were 3.9% to 4.7%.
Keywords derivative signal
processing; hydride generation; atomic absorption spectrometry; tin of environmental water
1 INTRODUCTION
Tin is one of the elements having biological, environmental and technological importance.
Hence, the developing of an easy, sensitive, precise method is desired for the
determination of trace tin.
In recent years, several analytical techniques and methods have been
used to determine total tin in environmental and biological samples. Yoshinaga et al
determined the total tin in sediment certified reference materials by isotope diluted
inductively coupled plasma mass spectrometry(ICP-MS) after alkali fusion [1].
The sensitivity of ICP-MS is the highest, however, ICP-MS is not an option method in the
determination of trace amounts of tin in complex matrices, because it would most likely
suffer from severe matrix interferences.
Many researchers prefer atomic absorption spectrometry, owing to its
simpler and less expensive instrumentation. Despite the sensitivity and accuracy inherent
in AAS, many analysts have found tin determinations to be troublesome[2]. In
the case of tin determination by GFAAS, the direct determination has been fraught with
problems, such as volatilization loss, interaction with the graphite tube, and vapor phase
interference[3]. For determination of trace tin in environmental and biological
samples, pre-concentration technique is generally required. Narukawa determined total tin
in environmental and geological samples by GFAAS using a tungsten furnace after
pretreatment by solvent extraction and cobalt(¢ó)
oxide collection[4]. Arpadjan et al determined Sn in water samples by
simultaneous inductively coupled plasma-atomic emission spectrometry(ICP-AES) and
electrothermal atomic absorption spectrometry(ETAAS) using preconcentration method with
dithiocarbamate loaded polyurethane as a sorption form[5].
As one of the few high sensitivity determination techniques, hydride
generation atomic absorption spectrometry(HGAAS) has become a popular method for the
determination of tin, since the analyte is separated from the matrix elements in solution.
Hydride generation had been used for determinations of tin by GFAAS[6]£¬
ICP-AES[7, 8 ], and DCP-AES[9]. Li G F[10]
determined tin by hydride generation atomic absorption spectrometry (HGAAS) with detection
limit of 0.76 mg·L-1. Le and Cullen described a new continuous hydride
generator for the determination of tin by HGAAS[11]. Tsuda et al determined
inorganic tin in biological samples by HGAAS after silica gel cleanup[12]. Fang
et al determined trace amounts of tin by flow-injection hydride generation atomic
absorption spectrometry with on-line ion-exchange separation and preconcentration[13].
Tin in natural waters is generally present at very low concentrations.
Quantitative analysis of trace tin requires a method that either has extremely lower
detection limit or involves a preconcentration step. Derivative measurement technique
coupling with hydride generation AAS (DHGAAS) had been developed for the direct
determination of selenium, tellurium and lead with very higher sensitivity and lower
detection limit without preconcentration[14-16].
The aim of this work is to establish a new method for the direct
determination of trace tin in water samples by HGAAS coupling with derivative signal
processing without pre-concentration. The proposed method was applied to the determination
of trace tin in water samples.
2 EXPERIMENTAL
2.1 Instrumentation
A Perkin-Elmer (USA) Model MHS-20 hydride generation bath system was
used. Ar was used as purge and carrier gas. The generated hydrides were atomized in
electrothermal quartz cell. All measurements were performed with a Model Zeeman 5000
atomic absorption spectrometer. Perkin-Elmer hollow cathode lamp (HCL) and recorder (Model
R 100A) were used.
The laboratory-made derivative system was connected between the atomic
absorption spectrometer and a double-pen recorder. The operating parameters and the
instrument settings were adjusted according to the manufacturer's recommendations. Table 1
lists the instrument settings. No background correction was used.
Table 1 Instrument
parameters and operating conditions
Resonance
wavelength (nm) |
286.3 |
Spectral band
(nm) |
0.7 |
Quartz
tube temperature (¡æ) |
950 |
Lamp current
(mA) |
8 |
Recorder
range (mV) |
5 |
Argon
pressure (kg·cm-2) |
2.5 |
Purge I
(sec) |
15 |
Reaction time
(sec) |
10 |
Purge II
(sec) |
60 |
2.2 Reagents
The tin stock solution, 1000mg·L-1,
was prepared by dissolving1.0000 g of metallic tin (high purity grade) in 100 ml of
concentrated hydrochloric acid and diluting to 1000ml with sub-boiling distilled water.
The working standard solutions were prepared daily by successive dilution of this solution
with 2% HCl.
The potassium tetrahydroborate stock solution, 10% (m/V), was prepared
by dissolving 10g KBH4 in 100ml 0.2 mol·l-1 sodium hydroxide. The solution was kept in a
refrigerator and was stable for up to four weeks. The working solution of potassium
tetrahydroborate was prepared daily by dilution of the stock solution with sub-boiling
distilled water. Boracic acid solutions was prepared by dissolving 40g H3BO3
in 1L of water.
All chemicals were of analytical-reagent grade unless specified
otherwise, and sub-boiling distilled water was used throughout the experiment. High-pure
argon was used as carrier gas.
2.3 Water samples and their characterizations
Four kinds of water samples were used for the determination of tin. These water samples
and their characterizations were described as follows.
2.3.1 Mineral water sample The mineral water for drinking was bought from
the market.
2.3.2 Waster water Waster water 1 was sampled from Fuhe river in Baoding
of Hebei Province. The waster water 2 was taken directly from a ditch near a factory. The
ditch water appeared to have come from surface runoff, although mixing with the
groundwater is possible before the water was discharged to the ditch. These samples were
filtered through 0.45mm
syringe filters using a micropore filter assembly. They were kept at 4¡ãC for a brief
period of time before analysis.
2.3.3 Tap water The tap water used in this study was taken from the
campus of Hebei University. The source of the water is Xidayang Reservoir of Hebei
province. The water sample was taken after running the tap for a few minutes and analyzed
without further treatment.
2.4 Recommended operating procedure
Dispensed an aliquot of standard solution, or 15 mL sample, into the reaction flask. Then,
15 ml 40g·L-1 H3BO3
or 0.6g solid H3BO3 was added into the flask to adjust the acidity.
1% (m/v) KBH4 in 1% NaOH was pumped. The generated hydride transported
immediately through a PTFE tube by a carrier gas stream into the quartz tube heated at 950¡æ. Derivative atomic absorption signal profile at 286.3nm was
recorded and the peak height was measured using the optimized DHGAAS procedure.
3 RESULTS AND DISCUSSION
3.1Mathematical model and characteristic of derivative signal
Derivative hydride generation atomic absorption spectrometric system was described in the
literature[14-16]. The conventional signals and derivative signals were
recorded by a double-pen recorder. The conventional signal of HG-AAS is pulse signal. The
derivative signal consists of up-peak and down-peak which correspond to the up-side and
down-side of the conventional signal. The relationship between the signal intensity and
time can be obtained by measuring absorbance of standard solutions at any time. The
mathematical model for lead signal had been obtained by simulating the measured signal[16].
Handled with the same method, the mathematical model of the derivative signals for Sn was
obtained as follows:
for up-peak: DH-U = m¡Á
B¡Á RC¡Á = m¡Á B¡Á RC¡Á A0¡Á ¡Áta-1 (1)
for down-peak: DH-D = m¡Á B¡Á RC¡Á = m¡Á B¡Á RC¡Á A0¡Á ¡Átc-1
(2)
Where a, b, c and d are constants within a large range of
concentration. a, b, c and d are 1.01, 7.14, 1.58, 24.56, respectively. m is the amount of
the amplification, RC is a time constant, and B is a constant related to the derivative
sensitivity.
Based on fundamentals of the derivative measurement system [16],
the heights of the up-peak and down-peak of the derivative signal can be expressed by:
DH=DH-U + DH-D = m¡Á B¡Á RC¡Á A0¡Á
( ¡Á ta-1 + ¡Á tc-1 )
(3)
Under the derivative measurement conditions selected, the equation can be reduced to:
DH=DH-U + DH-D =k(a+b)A0
(4)
Where a and b are constant
related to the analyte.
Because the absorbance (A0) is directly related to the
concentration in the solution(C), the equation can be expressed by:
DH=DH-U + DH-D =K(a+b)C (5)
For the selected conditions the total heights of the up-peak and down-peak are in direct
proportion to the concentration of tin.
3.2 Effect of acid medium
The influence of the concentration of hydrochloric acid and boracic acid on derivative
absorbance for tin was investigated, respectively. The experimental results were shown in
Fig. 1. The results suggested that the derivative absorbance for tin was higher in the
range of 0.05-0.1% HCl, and decreased markedly when the acidity higher than 0.1% HCl. In
weak acid medium of H3BO3, the sensitivity for tin was increased
about 3-4 fold. Derivative absorbance was higher in the range of 35-50g·L-1 H3BO3. Used H3BO3
as medium, the optimum acid range is broader and derivative absorbance is higher. Boracic
acid solution of 40g·L-1 was selected as optimum acid medium in this
work.
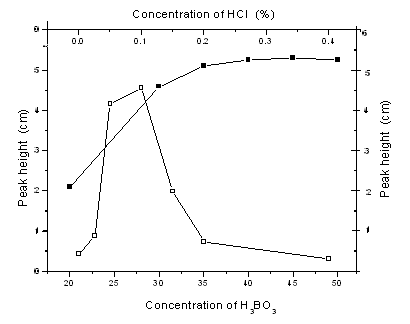
Fig. 1 Effect of HCl and H3BO3 concentration on the
peak height of Sn
¡ö20mL 0.2mgL-1 Sn (5 mVmin-1)
in medium of H3BO3; ¡õ60mL 0.2mg·L-1 Sn in medium of HCl
3.3 Effect of potassium
tetrahydroborate strength
Typical concentrations of potassium tetrahydroborate as reducing agent employed in HG-AAS
are less than or equal to 3%. Higher concentrations produce foam which result in signal
fluctuations. The effect of the concentration of KBH4 (in 1% NaOH) was tested
in the 0.5-3% KBH4 range. As a result, the maximum absorbance was obtained when
more than 1% KBH4 was added. In this investigation, 1% KBH4 in 1%
NaOH was used for a batch.
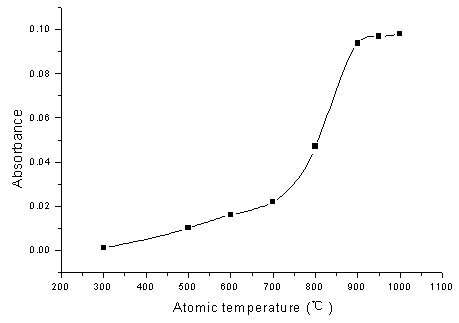
Fig. 2 Effect of atomic temperature on the absorbance of tin
3.4 Effect of atomization temperature
The effect of atomization temperature (from 300 ºC to 1000ºC) on the absorbance of Sn was examined. As shown in
Fig.2, atomic tin began to form at 500ºC.The peak height of the derivative signal
increased and the peak width of the derivative signal decreased sharply with the increase
of the atomization temperature from 700ºC to 900ºC.
The highest absorbance was obtained when the atomization temperature was above 900ºC.
The atomization temperature was set at 950ºC during the determination process.
3.5 Influence of the quartz surface on hydride atomization
It is evident and well documented that frequently used quartz cells are easily
contaminated for tin that may form a layer on the interior surface after a single
measurement [17]. It is usually necessary to clean the atomizer tube.
Otherwise, signal instability together with low absorbance signal for Sn are obtained. To
stabilize the signal, the treatment method (already tested by other authors) is the
immersion of the tube in a 40% HF solution for 15 min.
3.6 Interferences
A number of workers have investigated the interference effects in the determination of tin
by hydride generation in HCl medium. Stannane generation techniques can be affected by
interferences from transition metals, such as Ni2+, Co2+ and Cu2+[18].
Due to interferences from metals, the applicability of the method to real samples was
restricted. The use of L-cysteine to reduce interference in the hydride generation process
is strongly indicated.
In this work, interference of the transition metals on the
determination of tin (2ng) was studied in H3BO3 medium added with
different amount of Ni2+ as Ni(NO3)2, Co2+ as
Co(NO3)2 and Cu2+ as Cu(NO3)2.
50-1000 fold concomitant ions can be tolerated without interference in the absence of
L-cysteine. This result suggested anti-interference ability was increased.
3.7 Linearity, sensitivity, detection limits and precision
The linearity regression equations and correlation coefficients of DHGAAS at different
sensitivity grades for tin are listed in Table 2.
The calibration curves were linear in the concentration range 0f 0-80mg·L-1 for Sn with
correlation coefficients of 0.9983 to 0.9993.
Table 2 Regression equation
and correlation coefficient of DHGAAS
Derivative
grade (mV·min-1) |
Regression
equation |
Correlation
coefficient |
50 |
D=0.0512C*-0.0053 |
0.9992 |
20 |
D=0.1244C*+0.0037 |
0.9983 |
10 |
D=0.2477C*+0.0072 |
0.9993 |
5 |
D=0.5200C*-0.0021 |
0.9986 |
2 |
D=1.0200C*-0.0052 |
0.9990 |
* mg·L-1
The output signal of
the spectrometer was magnified and then differentiated by the derivative measurement
system. Effects of different derivative sensitivity grades on sensitivity, detection limit
and precision were studied with tin standard solution. The results show that the smaller
the number of derivative sensitivity grade (i.e., the higher the amplification), the
higher is the derivative absorption signal with the same solution. The derivative
sensitivity grade could be selected based on the concentration of the analyte.
The characteristic concentration expressed as sensitivity is defined as
the concentration which gives the derivative absorbance of 0.0044. The detection limit is
the concentration corresponding to triple the standard deviation of a series of eleven
measurements at blank level. The sensitivity, detection limits and precision of DHGAAS and
HGAAS are listed in Table 3.
It is apparent from these data that sensitivities were increased by
43-fold and detection limits were improved by 11-fold for Sn, respectively, as compared to
conventional HGAAS when using sensitivity range of 2 mV·min-1.
Table 3 The sensitivity,
detection limits and precision of D-HG-AAS and HG-AAS
Method |
Derivative
range (mV·min-1) |
Characteristic
concentration (mg·L-1) |
Detection
limits
(mg·L-1) |
RSD (%) |
HGAAS |
|
0.1833 |
0.2338 |
3.90 |
¡¡
DHGAAS |
50 |
0.0859 |
0.2593 |
3.32 |
20 |
0.298 |
0.1496 |
4.01 |
10 |
0.0178 |
0.0668 |
4.32 |
5 |
0.0085 |
0.0389 |
4.45 |
2 |
0.0042 |
0.0210 |
4.89 |
3.8 Application to practical samples
The practical feasibility of the proposed system was tested on three water samples. Since
standard reference materials with certified tin values were not available, the specificity
and accuracy of the method were tested by standard spiking method. The recoveries were
between 96% and 102%, it demonstrates the general reliability of the method.
Table 4 Concentrations of
tin in water samples
Sample |
Content of
Sn
(mg·L-1) |
Sn added
(mg·L-1) |
Sn found
(mg·L-1) |
Recovery
(%) |
RSD(%)
(n=7) |
Mineral
water |
0.080 |
0.050 |
0.130 |
100 |
4.4 |
Waster
water1 |
0.213 |
0.050 |
0.264 |
102 |
3.9 |
Waster
water2 |
0.104 |
0.050 |
0.153 |
98 |
4.7 |
Tap water |
0.45 |
0.10 |
0.053 |
96 |
4.5 |
4 CONCLUSION
The results show that H3BO3 medium is superior to HCl in many
technical aspects including sensitivity and anti-reference. The proposed method can be
used for direct determination of tin at sub-mg·L-1 level in environmental water.
REFRENCES
[1] Yoshinaga J, Nakama A, Takata K. Analyst, 1999, 124: 257.
[2] Dogan S, Haerdi W. Int J Environ Anal Chem, 1980, 8 (4): 249.
[3] Subramanian K S. Talanta, 1989, 36 (11): 1075.
[4] Narukawa T. J Anal At Spectrom, 1999, 14: 1081.
[5] Arpadjan S, Vuchkova L, Kostadinova E. Analyst, 1997, 122: 243.
[6] Meinrat O A, James T B. Analytica Chimica Acta, 1984, 156: 147.
[7] Christine Rigby, Ian D Brindle. J Anal At Spectrom, 1999, 14 (2): 253.
[8] Feng Y L, Chen H Y, Chen H W et al. Analytical and Bioanalytical Chem, 1998, 361 (2):
155.
[9] Brindle I D, Le X C. Analyst, 1988, 113: 1377.
[10] Li G F. Environmental Monitoring in China (Zhong Guo Huan Jing Jian Ce), 1999, 15
(6): 23.
[11] Le X C, Cullen W R. Analytica Chimica Acta, 1992, 258: 307.
[12] Tsuda T, Wada M, Aoki S. J Assoc Off Anal Chem, 1988, 71 (2): 373.
[13] Fang Z L, Sun L J, Hansen E H et al. Talanta, 1992, 39: 383.
[14] Sun H W, Ha J, Zhang D Q et al. Analytical Science, 2002, 18: 603.
[15] Ha J, Sun H W, Sun J M et al. Analytica Chimica Acta, 2001, 448: 145.
[16] Sun H W, Ha J, Sun J M et al. Fresenius J Anal Chem, 2001, 371 (8): 1154.
[17] Mayer D, Haubenwallner S, Komus W. Anal Chim Acta, 1992, 268: 315.
[18] Brindle I D, Le X C. Analyst, 1988, 113: 1377.
¡¡
|