Anovel synthesis of the
third-order optical nonlinearities of m, m' - diaminobenzophenone and
pyromellitic dianhyrtide polycondensate
Lu
Jianmei, Chen Naiyong, Ji Shunjun, Jia Zhun, Sun Zhenrong#,
Zhu Xiulin, Shi Weiping
(Chemisty Department of Suzhou University, Suzhou, Jiangsu Province; #Key
Laboratory of Optical & Magnetic Resonance Spectroscopy, East China Normal University
Department of Physics, East China Normal University)
Received Dec. 11, 2002; Supported by the National Natural Science Foundation of China
(No. 20076031) and Jiangsu Province Natural Science Foundation (No. BK2002042).
Abstract Polycondensation and imidization
of m, m'-diaminobenzophenone (m, m'-DABP) and pyromellitic dianhydride
(PMDA) under microwave radiation were studied, and the polycondensate's third-order
optical nonlinearities and time responses were measured by phase conjugate forward
three-dimensional degenerate four-wave mixing technique for the first time. The effect of
microwave radiation time (power), the composition of monomer and the temperature on the
imidization and the polymer yield were discussed. At the same time, the results of the
microwave radiation polymerization were compared with thermopolymerization. The results
showed that the polycondensate prepared had high third-order nonlinear optical
coefficients ( PAA c(3)
= 1.642×10-13esu) and fast time responses ( 24ps), simultaneously the
third-order optical nonlinearities of the polymer were improved greatly by microwave
radiation. The experimental results also showed that the third-order optical
nonlinearities were not only affected by the length of polycondensate chain, but also the
molecular structure, which was explained and confirmed by the computation of AMI
semi-empirical method, and UV visible absorption spectrum.
Keywords microwave, third-order optical nonlinearities, polyimide,
imidization
In recent years, there has been growing
interest in applying microwave energy to synthetic organic chemistry and synthetic polymer
chemistry as well. Since the appearance of first article on the use of microwave energy in
chemical synthesis, the approach has now developed into a useful technique for a variety
of application in organic synthesis, especially, the solventless reactions conducted on
solid supports[1]. In the field of synthetic polymer chemistry, microwave
energy has been utilized for the radical polymerization of vinyl monomers such as
2-hydroxy ethyl methacrylate[2], methyl methacrylate[3], and styrene[4],
and for the curing of polymers such as epoxy resins[5-11] and polyurethanes[12-13],
as well as for the imidization of polyamic acids[14-15]. In most cases, the
high heat efficiency gave rise to remarkable rate enhancement and dramatic reduction of
reaction time. Recently, the rapid synthesis of aromatic polyamides by microwave-assisted
direct polycondensation of aromatic diamines and aromatic dicarboxylic acids with
condensing agents[16-17], and a preliminary study on the microwave-assisted
rapid synthesis of polyamides from nylon salts have been intensively investigated by Imai
et al.[18]. However, there is no report so far on the synthesis of
benzoguanamine and 2,4-tolylenediisocyanate by using microwave energy except for our
studies. During the course of our studies, we have already reported the microwave
irradiation solid state copolymerization in binary maleic anhydride and dibenzyl maleate
system[19], copolymerization of maleic anhydride and allyl thiourea in solid
state. In polycondensation field microwave irradiation were widely applied. Since
polyimides are important as photochemical materials in the third-order optical
nonlinearities. So we have tried to afford useful products by using method of microwave
irradiation. Herein, we report that the polymeriztion of m, m'-diaminobenzophenone
(m, m'-DABP) and pyromellitic dianhydride (PMDA) and imidization of m, m'-DABP-PMDA
polycondensate (PAA) under microwave irradiation. Moreover, the third-order optical
nonlinearities of the polymers are discussed.
1.EXPERIMENTAL
1.1 Experimental Setup
 
Scheme 1
Scheme 2
Scheme 1:Setup of solid phase
polymerization under microwave irradiation
Scheme 2 Setup of solution polymerization under microwave irradiation
Note: Reaction temperatures are measured by alcohol thermometer
1.2 Procedure of Polymerization
m, m'-Diaminobenzophenone (m, m'-DABP) were synthesized according to the
literature, purity 98.5%; Pyromellitic Dianhydride (PMDA), purity≥98%(Shanghai Jiaohua Factory), vacuum dry.
(1) Solution polymerization. m, m'-DABP were dissolved in DMF 10 minutes under
nitrogen flow. After completely dissolved, PMDA (m,m'-DABP:PMDA(mol)=1:1)were added in
batches. The temperature was kept at 45 ºC
by controlling the power. Temperature was measured by means of setting alcohol thermometer
into the polymerization system, and the reaction time was kept for 2 hours. PAA were
produced and precipitated by pouring distilled water, and washed by methanol.
(2) Solid phase polymerization under microwave radiation. PAA powders were set into the
reactor under N2, and dehydrated PAA was for imidization under continuous
microwave radiation. Temperatures were measured by means of setting alcohol thermometer
into the polymerization system.
(3) Solid phase thermopolymerization. PAA powders were set into the reactor under N2,
and dehydrated PAA at the temperature, which was the same as the maximal temperature of
solid phase polymerization under microwave radiation.
1.3 Measurement
The confirmation of structure of the polymers was confirmed by means of IR and H-NMR.
H-NMR (D-DMF): 7.44-8.34ppm (=CH-), 10.80 ppm (–COOH), 6.85ppm(–NHCO–).
IR(KBr): 1651cm-1 ( in the –NHCO–), 1255cm-1 (–C–N–), 1780 cm-1, 1725cm-1( in the ).
The intrinsic viscosity of polymer were measured by extrapolation in
the solvent: DMF at 30+1ºC.
The imidization degree of PI were measured by using Infrared absorption
spectrum that Masatoshi Hasegawa[20] had used. The stretch vibration of C=O of
polyimide were expressed as the imidization degree by using the stretch vibration of C=C
in benzene ring as the reference.
The third-order optical nonlinearities of polymer were measured by
phase conjugate forward three-dimensional degenerate four-wave mixing (3D DFWM) technique
(in Department of Physics, East China Normal University).
2. Results and Discussion
2.1 Synthesis of polymer
This polymer was synthesized by polycondensation of m, m'-DABP and PMDA in
two-step. Firstly, PAA polymer was got by polycondensation of m, m'-DABP and PMDA,
then the polymer(PI) was obtained by imidization of PAA. In this polymerization, microwave
irradiation and regular heating methods were used. The procedure of polymerization was
shown in Scheme 3.
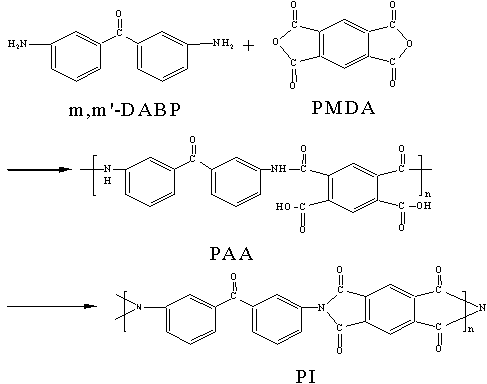
Scheme 3 The procedure of polymerization.
2.2 The third-order optical nonlinearities of
m, m'-DABP-PMDA polymer
The third-order optical nonlinearities of the m, m'-DABP-PMDA polycondensate were
measured by phase conjugate forward three-dimensional degenerate four-wave mixing (3D
DFWM) technique[29-30] as depicted in Figure1. After passing through a quarter
wavelength plate, the pulse laser beam (wavelength 532 nm, pulse-width 15ps, repetition
rate 10Hz, peak irradiance 10GW/cm2), from a frequency-doubled picosecond pulse
mode-locked Nd:YAG laser, is split into three beams k1, k2 and k3
with the same energy by use of reflecting beam splitters, then temporally and spatially
overlapped in the sample with a 205mm focal-length lens. The angles between the three
beams k1, k2 and k3 are about 1o. In the
experiment, the intensity I4 of the phase conjugate beam k4 is
detected by PIN photodiode. According to the following formula, the third-order nonlinear
optical coefficients c (3) can be measured by compared the measured signals for
solution with that for carbon disulfide as reference under the same experimental
condition:
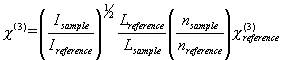
Where I is the intensity of signal peak, L is the thickness of the sample, n is the
refractive index, the subscript r refers to carbon disulfide, and the tensor elements c(3)xxxx
= 6.8×10-13 esu and c(3)yxxy = 7.6×10-14
esu for carbon disulfide. The tensor elements of third-order optical nonlinearities
for m, m'-DABP-PMDA polycondensate are calculated.
2.3 The setup for measuring the third-order optical nonlinearities of the polymer
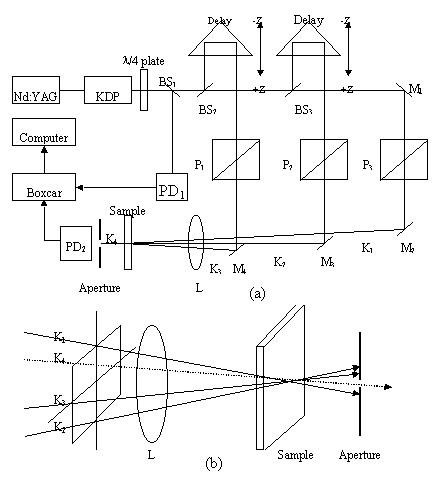
Figure1 The 3D DFWM setup for measuring the
third-order optical nonlinearities of the Polymer
(a) The experimental setup for three dimensional DFWM
(b) Configuration for three dimensional DFWM
2.4 The mechanism of the third-order optical
nonlinearities
In this paper, the mechanism of the third-order optical nonlinearities of polycondensate
were studied by AM1 (AM1 means Austin mode one) semi-empirical method, UV-Visible
absorption spectrum and the time response spectrum for the DFWM signal.
2.4.1 The charge density
In order to study the p-p -conjugated structure of m, m'-DABP-PMDA polycondensate
(PAA) and their imidization polymer (PI), the charge densities were calculated by the
computation of AMI semi-empirical method. The results were shown in Figure 2.
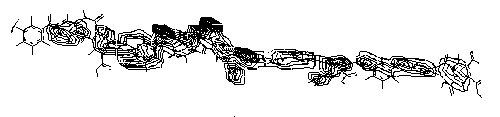
a. PAA

b. PI
Figure 2 The charge density of m, m'-DABP-PMDA polycondensate
The result showed that
the polycondensate were the p-p -conjugated system.
2.4.2 The UV-Visible absorption spectrum
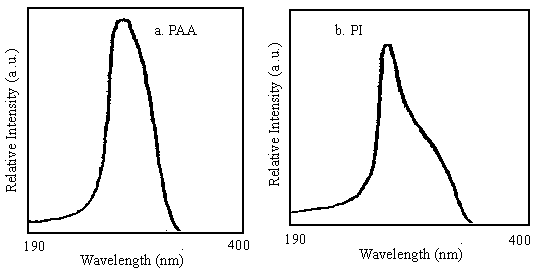
Figure 3 The UV-visible absorption spectra of m, m'-DABP-PMDA
polycondensate
The UV-Visible
absorption spectrums of m, m'-DABP-PMDA polycondensate are shown in Figure 3, where
the absorption bands in the region of 230-350 nm of PAA and 236-355 nm of PI are due to p
-p * transitions of the highly polyconjugated systems, indicative of the
distribution range in the p-p -conjugated systems, and the used laser wavelength (532nm)
in the experiment is out of the absorption region.
2.4.3 The time response spectrum for the DFWM signal
m, m'-DABP-PMDA polycondensate, with a large quasi-one-dimensional p-p -conjugated
structure, contain a high density of p -electron that attributed to the third-order
nonlinearities. A delay for any one of the three input beams gives almost the same signal
envelope. The time-resolved measurement results of 3D DFWM are shown in Figure 4. The
envelope of the signal is fitted very well by a Gaussian function, and the half-width of
the fitting envelope (about 25ps) is similar to the autocorrelated pulse duration. The
signal profile shows the symmetry about the maximum signal (the zero time delay), which
indicates that the response time (in Table 1) of the third-order optical nonlinearity is
equivalent to experimental time resolution (PI=25ps). It means that the time response of
the third-order optical nonlinearity is quite fast, and the Kerr effect, which arises from
the distortion of the large p-p -conjugate electronic charge distribution of m, m'-DABP
-PMDA polycondensate, is the main reason for generating DFWM.
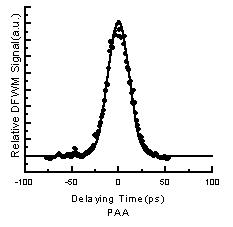 
Figure4 The DFWM time response spectra for m,m,-DABP-PMDA polycondensate
Table1 The time response for the DFWM
signal of the m,m,-DABP-PMDA polycondensate
Sample |
Response time (ps) |
m,m,-DABP-PMDA PAA |
27 |
m,m,-DABP-PMDA PI |
25 |
2.5 The effect factors of the third-order
optical nonlinearities of the polymer
2.5.1 Effect of the conjugated chain length
The relationship between the third-order optical nonlinearities and the intrinsic
viscosity of polymer were shown in Figure 5(varying the microwave radiation energy) and
Figure 6 (varying the monomer composition). It can be learned that the third-order
nonlinear optical coefficient c(3)
increase as the intrinsic viscosity of polymer (the conjugated length L ) increases in
this experimental range. It is known that when the wavelength of strong laser field is the
region of materials absorption, the kerr nonlinear effect of resonant strengthening will
be produced. On this condition, the molecules are approximately regarded as two energy
level system. The nonlinear polarization is relative to the molecular energy level of
excitation, especial inverse proportion to the energy gap between the LUMO (Lowest
unoccupied molecule orbit) and the HOMO (Highest occupied molecule orbit).
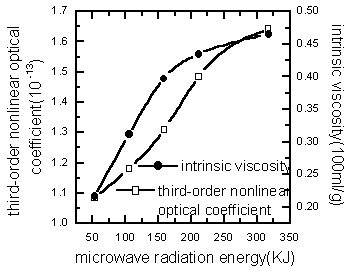
Figure 5 Relationship among
third-order nonlinear optical coefficient of PAA and intrinsic viscosity with the
microwave energy
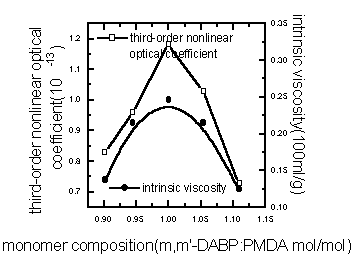
Figure 6 Relationship among third-order
nonlinear optical coefficient of PAA and intrinsic viscosity with the monomer composition
The energy gap Eg values
for the various degree of polymerization (n=1-4) conjugated lengths of polymer are
calculated by AMI semi-empirical method (see Table 2). The Eg decreases as the
conjugated length of polymer chain increases. It may be that the increase of the
conjugated degree results in the decrease of the Eg value. As a result, the
conjugated electrons generate transition quickly and the distortion of conjugated
electrons can come into being easily. The results show that the third-order optical
nonlinearities of conjugated polymer increase as the length of p-p -conjugated structure
increase.
Table2 The calculated energy gap Eg for
the various conjugated lengths of the polymer by AM1 semi-empirical method
Sample |
Eg(ev) |
n=1 |
n=2 |
n=3 |
n=4 |
m, m'-DABP-PMDA PAA |
6.152 |
5.711 |
5.675 |
5.587 |
m, m'-DABP-PMDA PI |
5.921 |
5.895 |
5.871 |
5.869 |
2.5.2 Effect of microwave radiation and
heating on the solution polymerization ( polycondensation of m, m'-DABP and PMDA)
From Figures 7-9, it can be learned that the third-order nonlinear optical coefficient c(3) and the intrinsic viscosity of PAA, which are synthesized
by microwave radiation or heating, increase as the temperature increase, then decrease as
the temperature go on increasing. The c(3)
of PAA which are synthesized by microwave radiation is larger than that by heating. It may
be that the microwave radiation contributed to the narrow distribution of molecular
weight. The result showed that microwave radiation could improve the third-order optical
nonlinearities of polymer, and took on the unheating effect, which may have a particularly
active action.
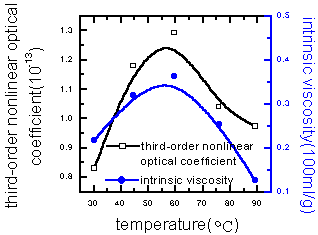 |
|
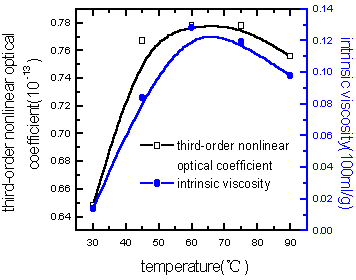 |
Figure 7 Relationship among third-order nonlinear optical coefficient of PAA
synthesized in the solution by microwave radiation and intrinsic viscosity with the
temperature |
|
Figure 8 Relationship among third-order nonlinear optical coefficient of PAA
synthesized in the solution by heating and intrinsic viscosity with the temperature |

Figure 9 Comparision the third-order nonlinear optical coefficient of PAA
synthesized in the solution by microwave radiation with by heating |
|

Figure10 Relationship between third-order
nonlinear optical coefficient of PI synthesized in the solid phase by microwave radiation,
the time and imidization degree |
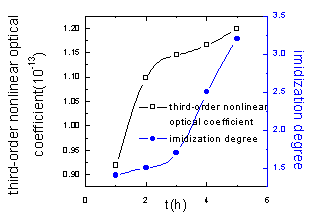 |
|
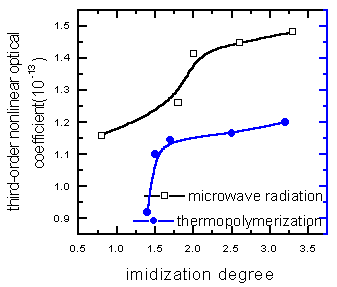 |
Figure11 Relationship among
third-order nonlinear optical coefficient of PI synthesized in the solid phase by heating,
the time and imidization degree |
|
Figure12 Comparision the
third-order nonlinear optical coefficient of PI synthesized in the solid phase by
microwave radiation with that by heating |
2.5.3 Effect of microwave radiation and
heating on the solid phase polymerization (imidization of PAA)
From Figure 10 to 12, it can be learned that the third-order nonlinear optical coefficient
c(3) of PI, which were synthesized in solid phase by microwave
radiation or heating, increase as the imidization degree increase. The c(3) of PI
which were synthesized in solid phase by heating is smaller than by microwave radiation.
It showed that microwave radiation can improve the third-order optical nonlinearities of
polymer, and took on the unheating effect, which may have a particularly active action.
REFERENCES
[1] Gedye R, Smith F, Westaway Ali K H et al. Tetrahedron Lett., 1986, 27: 279.
[2] Teffal M, Gourdenne A. Eur.Polym.J., 1983, 19: 543.
[3] Al Dori D, Huggett R, Bates J F et al. Dent. Matter., 1988, 4: 25.
[4] Stoffer J O, Sitatram S P. Am. Chem. Soc., Proc. Polym. Mater.Sci.Eng. , 1994, 71: 55.
[5] Mijovic J, Jawa J. Polym. Compos., 1990, 11: 184.
[6] Thuillier F M, Jullien H, Grenier-Loustalot M F. Polym.Commun., 1986, 27: 206.
[7] Lewis D A, Hedrick J C, McGrath J E et al. Polym.Prer., Am.Chem.Soc., Div. Polym.
Chem., 1987, 28 (2): 330.
[8] Van Q, Grourdene A. Eur. Polym. J., 1987, 23: 777.
[9] Beldjoudi N, Bouazizi A, Douibi D et al. Eur.Polym.J., 1988, 24: 49.
[10] Singer S M, Jow J, Delong J D et al. SAMPE Quat., 1989, 20: 14.
[11] Mijovic J, Wijaya J. Macromolecules, 1990, 23: 3671.
[12] Silinski B, Kuzmycz C, Grourdene A. Eur.Polym.J., 1987, 23: 273.
[13] Jullien H, Valot H. Polymer, 1985, 26: 506.
[14] Lewis D A, Summers J D, Ward T C et al. J. Polym. Sci., A. Polym. Chem., 1992, 30:
1647.
[15] Kishanprasad V S, Gedam P H. J. Appl. Polym. Sci., 1993, 50: 419.
[16] Park K H, Watanabe S, Kakimoto M et al. Polym. J., 1993, 25: 209.
[17] Watanabe S, Hayama K, Park K H et al. Makromol.Chem., Rapid Commun., 1993, 14: 481.
[18] Imai Y, Polym. Prepr. Am. Chem.Soc., Div. Chem., 1995, 36 (1): 711.
[19] Ji S J, Yu H, Lang J P et al. Chin. J. Struct. Chem.,(in Eng.)1997, 16: 290.
[20] Maloney C, Byrne H, Dennis W M et al. Chem. Phys., 1988, 121: 21.
一种具有三阶光学非线性性能材料-m, m'二氨基二苯酮与均苯四酸二酐的缩聚物的新型合成方法
路建美, 陈乃勇, 纪顺俊,朱健,孙真荣#,朱秀林, 施卫平
(苏州大学化学化工学院,江苏 215006; #华东师范大学物理系,光学磁共振波谱国家重点实验室,上海200000)
摘要 本文研究了m, m'二氨基二苯酮与均苯四酸二酐微波辐射下的缩聚及其亚酰化,并且使用前向相位共轭三维简并四波混频技术测定了接枝聚合物的三阶非线性光学性能和时间响应。分别讨论了微波辐射时间(功率)、单体组成以及聚合温度对于亚酰化度和产率的影响,同时将微波辐射得到的结果与常规加热聚合得到的结果进行比较。表明微波得到的缩聚物具有较高的三阶非线性光学系数(c(3)
= 1.642×10-13esu)和较快的时间响应(24ps),这说明微波辐射得到的缩聚物三阶光学非线性性能得到了提高。本文还通过使用AMI半经验计算的方法和紫外可见吸收光谱证实了缩聚物的链长和分子结构对三阶光学非线性性能有着一定的影响。
关键词 微波,三阶光学非线性,聚酰亚胺,亚酰化
|