Representation of ternary
liquid-liquid equilibria including two associating components in terms of
associated-solution model
Chen Yao*, Kazuhiro Tamura, Toshiro Yamada
(Department of Chemistry and Chemical Engineering, Division of Physical Sciences, Kanazawa
University, 40-20, Kodatsuno 2-chome, Kanazawa, Ishikawa 920-8667, Japan;
*Jointed with the Department of Chemistry, College of
Life Science and Technology, Jinan University, Guangzhou, 510632, China )
Received Apr. 30, 2002.
Abstract Two solvation
models, single and multi-solvation forms of an extended UNIQUAC associated-solution model,
are presented to correlate binary vapor-liquid equilibria and mutual solubilities,
furthermore, are employed to predict ternary liquid-liquid equilibria for mixtures
including alcohol, acetonitrile and aniline. The models considering reasonably
intermolecular association and solvation formed by hydrogen-bonding molecules are able to
explain well the thermodynamic properties of multicomponent mixtures. Good calculated
results demonstrate that the two solvation models are successful in the accurate
representation of binary vapor-liquid and liquid-liquid equilibria as well as ternary
liquid-liquid equilibria systems consisting of two associating molecules.
Keywords Vapor-liquid equilibria, Liquid-liquid equilibria, Ternary
mixtures, Associated-solution model
1. INTRODUCTION
Hydrogen-bonding molecules such as alcohol, acetonitrile and aniline can interact possibly
to form chemical complexes through the association and solvation between like and unlike
molecules. The chemical complex formation is not easily characterized, but various
procedures and models have been applied to understand these properties. As a whole
thermodynamic properties of these mixtures can provide the useful information of the
molecular interactions, and the thermodynamic models related to the molecular properties
are often used to interpret the bulk properties so far. The results can be often model
dependent and sensitive to the assumptions of the model, but the description of
liquid-liquid equilibria (LLE) for ternary mixtures of alcohol, acetonitrile and aniline
is one of the most powerful probe for the thermodynamic models to account for the chemical
complex formation between these hydrogen-bonding molecules.
An extended form of the UNIQUAC associated-solution model[1]
taking into account the molecular association and solvation between the component
molecules was proposed in a previous work to represent accurately the phase equilibria of
multicomponent mixtures including one of alcohols, acetonitrile and aniline with
non-associating components and the model shows an excellent performance in correlating
binary vapor-liquid equilibria (VLE) and mutual solubilities as well as in predicting
ternary LLE. Especially this extended model including the association and solvation of
acetonitrile improved more successfully the representation of the ternary LLE for the
mixtures of acetonitrile with a non-associating component than the UNIQUAC
associated-solution model of Nagata.[2]
Accordingly, in order to represent ternary LLE for the mixtures formed
by two associating molecules of alcohol, acetonitrile and aniline with one non-associating
molecule, we assume two solvation models coupled with the extended UNIQUAC
associated-solution model and aim at obtaining an understanding of the behavior for these
mixtures as well as an accurate description of the ternary LLE. The experimental phase
equilibrium data for the binary and ternary mixtures containing alcohol, acetonitrile and
aniline examined are available from the literature.
2. EXTENDED UNIQUAC ASSOCIATED-SOLUTION
MODEL
The extended UNIQUAC associated-solution model [1] was proposed previously for
phase equilibria of the multicomponent mixtures containing one alcohol and non-associating
component.
Associating components A and B self-associate to form
linear polymers according to the following successive reactions:
(1)
(2)
and the association equilibrium constants characterized in terms of the corrected segment
fractions of the chemical species existed in the mixtures, are defined by
(3)
(4)
K is the association equilibrium constant and independent of the degree of
association, and is dependent on temperature and K° is the values of K at
T = 323.15K. h is the enthalpy of hydrogen-bond formation and is assumed to be
independent of the degree of association and of temperature.
The associating molecules
and can solvate each other. Two solvation
models, named by single solvation and multi-solvation are considered in the following
sections.
2.1 Single solvation model
The associating molecule species, Ai and Bj, indicated
A = alcohol, B = acetonitrile or aniline, solvate each other to form species
AiBj in the following manner:
(5)
where the suffixes i and j range from unity to infinity. The solvation
constant for the above reaction is expressed by
(6)
The activity coefficient of associating component i (i = A or B)
is given by
(7)
The activity coefficient of non-associating component C is given by
(8)
where the modified volume parameter, is set
to be , segment fraction, , the modified segment fraction, and the surface fraction, , are expressed as follows:
, ,
(9)
and the adjustable binary parameter, tji, is defined by
(10)
The modified area parameter is fixed to
be in the model. The modified segment
fraction of the monomer of associating component, and are obtained by solving
the mass balance equations for two associating components given by
(11)
(12)
The true molar volumes of pure component and ternary mixture, VA 0',
VB0', V ', are individually expressed by
(13)
(14)
(15)
The modified segment fraction of monomer of the self-associating component at the
pure-liquid state, and , can be expressed by
(16)
(17)
and for component C.
When specially KB=0, the single solvation model is
also employed to calculate the binary mixtures containing one associating component and
one non-associating component.
2.2 Multi-solvation model
The pure i-mers and j-mers of two alcohols molecules, A and B,
take place successive solvation step by step to form linearly polymer complex
multimers (AiBj)k, Ai(BjAk)l,
(BiAj)k and Bi(A jBk)l.
(18)
(19)
......
(20)
(21)
where the indices i, j, k and l can be any integers from 1 to infinity. We
assume that one solvation constant holds
for all above solvation reactions. The modified segment fractions of monomer of
associating components, and , are obtained from the following
mass balance equations:
 (22)
 (23)
where the sums , ,
and are defined by
(24)
(25)
(26)
(27)
The true molar volume of ternary mixture, V ', is expressed by
(28)
Table 1
Association constants and structural parameters for pure component
Component |
KA0(323.15K) |
-hA(kJ.mol-1) |
r |
q |
q' |
Acetonitrile |
7.0 |
6.7 |
1.50 |
1.40 |
q0.1 |
Aniline |
15.0 |
15.4 |
2.98 |
2.38 |
q0.1 |
1-Butanol |
69.5 |
23.2 |
2.77 |
2.42 |
q0.1 |
Cyclohexane |
|
|
3.18 |
2.55 |
q0.1 |
Ethanol |
110.4 |
23.2 |
1.69 |
1.55 |
q0.1 |
n-Heptane |
|
|
4.15 |
3.52 |
q0.1 |
n-Hexane |
|
|
3.61 |
3.09 |
q0.1 |
Methanol |
173.9 |
23.2 |
1.15 |
1.12 |
q0.1 |
1-Propanol |
87.0 |
23.2 |
2.23 |
1.98 |
q0.1 |
2-Propanol |
49.1 |
23.2 |
2.23 |
1.98 |
q0.1 |
r and q were calculated by the
method of Vera [7].
q' was fixed in this work.
3. CALCULATION PROCEDURE
The association constants of aliphatic alcohols at 50oC were
taken from Brandani [3]: methanol, 173.9; ethanol, 110.4; 1-propanol, 87.0;
2-propanol, 49.1; 1-butanol, 69.5. A value of hA=
-23.2 kJ·mol-1 taken from Stokes and Burfitt [4] was used as the
enthalpy of hydrogen-bonding alcohol molecules and assumed independent of temperature. The
association constant of acetonitrile [1] and aniline [5] is obtained
from the previous papers. The enthalpy of hydrogen bond formation in pure aniline [5]
was set as hA= -15.4 kJ·mol-1. The enthalpy of acetonitrile was taken from the
reference [1, 6]. Pure component structural parameters, r and q
were calculated by the method of Vera et al. [7], listed in Table 1.
Binary experimental VLE and LLE data reduction were performed according
to the following thermodynamic relations:
(29)
(30)
where P is the total pressure, x is the liquid phase mole fraction, y
is the vapor phase mole fraction, is the activity coefficient of component i, Pi0
is the vapor pressure of pure component i, was calculated using the Antoine
equation. V0 is the pure liquid molar volume, was obtained by a modified
Rackett equation [8]. is
the fugacity coefficient, was calculated by the eqn. (30), the pure and cross second
virial coefficients B were estimated by the method of Hayden and O'Connell [9].
A computation program based on the maximum likelihood principle
described by Prausnitz et al [10] was used to obtain an optimum set of the
model energy parameters by minimizing the objective function:
(31)
where the subscripts cal and exp individually indicate the most probable
calculated value corresponding to each measured point and experimental value. The standard
deviations in the measured variable were taken as: σP = 1 Torr,σT = 0.05 K, σx = 0.001 and σy = 0.003. σP for pressure, σT for temperature, σx for liquid-phase mole fraction, σy for vapor-phase
mole fraction.
The energy parameters for partially miscible mixtures at the indicating
temperature were obtained by solving thermodynamic eqns. (32) and (33) simultaneously.
(32)
and (33)
where superscripts, I and II, indicate two liquid phases.
Table 2 Solvation equilibrium constants and enthalpies of complex formation used
by the single solvation model
System (A+B) |
KAB(323.15K) |
-hAB(kJ.mol–1) |
Acetonitrile +
1-butanol |
15. |
17.0 |
Acetonitrile +
ethanol |
16. |
17.0 |
Acetonitrile +
methanol |
16. |
17.0 |
Acetonitrile +
1-propanol |
15. |
17.0 |
Acetonitrile +
2-propanol |
15. |
17.0 |
Ethanol +
aniline |
17.5 |
17.0 |
Table 3 Solvation
equilibrium constants and enthalpies of complex formation used by the multi-solvation
model
System (A+B) |
KAB(323.15K) |
-hAB(kJ.mol–1) |
Methanol + ethanol |
130 |
23.2 |
Methanol + 1-propanol |
120 |
23.2 |
Methanol + 2-propanol |
90 |
23.2 |
4. CALCULATED RESULTS
4.1 Binary vapor-liquid equilibria and liquid-liquid equilibria
The solvation equilibrium constant, listed in Tables 2 and 3, estimated by fitting the
single and multi-solvation models to reduce well binary VLE and decrease with the
increasing the number of carbon atoms in the alcohol. The enthalpies of complex formation
were approximately estimated by taking the difference between the enthalpy of infinite
dilution of ethanol in saturated hydrocarbons and that of ethanol in active solvents and
were assumed to be independent of the number of carbon atoms in alcohol molecules. Table 4
presents the calculated results by using the single solvation model for the 14 binary VLE
systems and for the 8 binary LLE systems formed by alcohol, acetonitrile, aniline, whose
data are available from the literatures [11-28].
Table 4 Calculated
results of binary phase equilibrium data reduction by using the single solvation model
System
(1+2) |
Temp.
/K |
Na |
a12
/K |
a21
/K |
dP
/kPa |
dT
/K |
dx
×103 |
dy
×103 |
Ref. |
Acetonitrile+
1-butanol |
333.15 |
8 |
-54.19 |
646.66 |
0.29 |
0.1 |
0.7 |
5.2 |
[11] |
Acetonitrile+
ethanol |
313.15 |
14 |
59.45 |
265.32 |
0.10 |
0.0 |
0.8 |
4.5 |
[12] |
Acetonitrile+
cyclohexane |
298.15 |
MSb |
157.12 |
465.48 |
|
|
|
|
[13] |
Acetonitrile+
n-heptane |
298.15 |
MS |
109.63 |
447.37 |
|
|
|
|
[14] |
Acetonitrile+
n-hexane |
298.15 |
MS |
103.19 |
455.73 |
|
|
|
|
[14] |
Acetonitrile+
methanol |
298.15 |
10 |
-15.89 |
110.59 |
0.10 |
0.0 |
0.9 |
6.0 |
[14] |
Acetonitrile+
1-propanol |
318.15 |
9 |
35.46 |
423.88 |
0.13 |
0.0 |
0.5 |
4.4 |
[15] |
Acetonitrile+
2-propanol |
323.15 |
15 |
412.13 |
166.92 |
0.05 |
0.0 |
0.4 |
2.8 |
[16] |
Aniline+
ethanol |
313.15 |
13 |
71.82 |
24.72 |
0.04 |
0.0 |
0.1 |
1.4 |
[17] |
Aniline+
cyclohexane |
298.15 |
MS |
371.57 |
104.54 |
|
|
|
|
[18] |
Aniline+
n-heptane |
298.15 |
MS |
469.60 |
179.53 |
|
|
|
|
[19] |
1-Butanol+
n-heptane |
333.15 |
19 |
243.88 |
-37.61 |
0.10 |
0.0 |
0.5 |
4.6 |
[20] |
Ethanol+
cyclohexane |
293.15 |
7 |
184.98 |
25.91 |
0.09 |
0.0 |
0.2 |
2.8 |
[21] |
Ethanol+
n-heptane |
303.15 |
22 |
164.41 |
53.37 |
0.05 |
0.0 |
0.1 |
|
[22] |
Methanol+
cyclohexane |
298.15 |
MS |
141.41 |
82.31 |
|
|
|
|
[23] |
Methanol+
n-heptane |
298.15 |
MS |
148.50 |
89.21 |
|
|
|
|
[24] |
Methanol+
n-hexane |
298.15 |
MS |
166.09 |
75.53 |
|
|
|
|
[24] |
1-Propanol+
cyclohexane |
298.15 |
27 |
326.47 |
-67.00 |
0.08 |
0.0 |
0.1 |
|
[25] |
1-Propanol+
n-heptane |
298.15 |
14 |
16.41 |
156.01 |
0.03 |
0.0 |
0.0 |
|
[26] |
1-Propanol+
n-hexane |
298.15 |
25 |
350.82 |
-30.01 |
0.08 |
0.0 |
0.1 |
|
[25] |
2-Propanol+
|cyclohexane |
323.15 |
9 |
225.25 |
-40.43 |
0.11 |
0.0 |
0.5 |
4.3 |
[27] |
2-Propanol+
n-hexane |
328.21 |
24 |
241.86 |
-23.54 |
0.20 |
0.1 |
2.9 |
4.1 |
[28] |
a Number of data points; b
Mutual solubilities.
Table 5 presents the
calculated results derived from the multi-solvation model for the 3 binary VLE systems
formed by two alcohols molecules, whose data are available from the literatures [29-31].
The calculated results shown in Table 5 were compared with those of the UNIQUAC
associated-solution model of Nagata.[2] The present model works better than the
Nagata model.
Table 5 Calculated
results of binary phase equilibrium data reduction by using the multi-solvation model
System
(1+2) |
Temp.
/K |
Na |
a12
/K |
a21
/K |
d P
/kPa |
d T
/K |
d x
×103 |
d y
×103 |
Ref. |
Methanol+Ethanol |
298.15 |
11 |
362.76 |
-173.06 |
0.03b
0.39c |
0.0
0.0 |
0.0
1.7 |
4.8
10.9 |
[29] |
Methanol+1-propanol |
333.15 |
24 |
-97.01 |
256.68 |
0.05
b |
0.0 |
0.3 |
1.4 |
[30] |
Methanol+2-propanol |
328.15 |
20 |
-122.33 |
261.68 |
0.18
b
0.49c |
0.1
0.1 |
0.9
2.2 |
4.2
11.6 |
[31] |
a Number of data points; b
Extended UNIQUAC associated-solution model; c UNIQUAC associated-solution
model.
4.2 Ternary liquid-liquid equilibria
The binary parameters, shown in Tables 4 and 5, obtained from the binary phase equilibrium
data reproduction were used to predict ternary LLE for many representative ternary
mixtures without any additional ternary parameters. We used the binary
parameters that were obtained from the experimental binary data at the temperature as
close as the ternary ones. The binary parameters obtained slightly depend on the
temperature, but the model can predict well the ternary LLE using the
temperature-independent binary parameters listed in Tables 4 and 5. The ternary
predicted results and experimental values were plotted in Figures 1 and 2 for some ternary
systems. Figure 1(a) for type II systems, where two binaries are partially miscible and
the third binary is completely miscible; Figure 1 (b) to (d) and Figure 2 (a) to (c) for
type I systems, where only one binary is partially miscible. Fig. 1 shows the ternary LLE
systems of one alcohol and one acetonitrile or aniline mixtures predicted by the single
solvation model having the only binary parameters taken from Table 4. Fig. 2 shows the
ternary LLE systems of two alcohol mixtures predicted by the multi-solvation model using
the binary parameters given in Table 5. The experimental tie-line data for the ternary
systems investigated here are available from the literatures [13,14,32-34].
Furthermore Figure 1 and 2 shows comparison of the predicted results of the extended
UNIQUAC associated-model with those of the UNIUAC model. Good agreement between the
results calculated by the present model for the ternary LLE with the experimental ones was
obtained, as demonstrated in Figures 1 and 2.
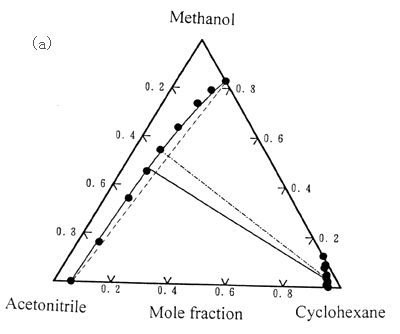
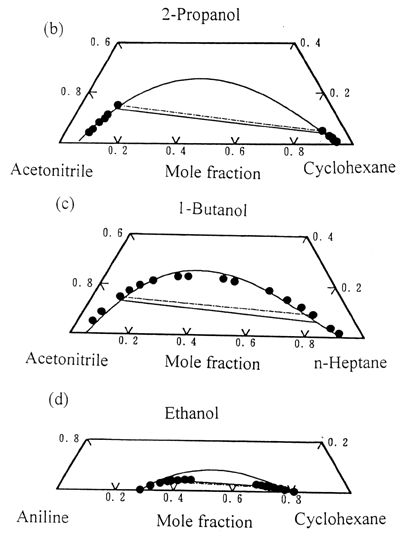
Fig.1 Liquid-liquid
equilibria for the ternary systems consisting of one alcohol and one acetonitrile or
aniline mixtures; Experimental tie-line: ● - ●; Predicted lines: ——,
Extended UNIQUAC associated-solution model; - - - - - , UNIQUAC model.
(a). Acetonitrile + methanol + cyclohexane at 25oC [14];(b).
Acetonitrile + 2-propanol + cyclohexane at 25oC [32];(c).
Acetonitrile + 1-butanol + n-heptane at 25oC [14]; (d). Aniline + ethanol + cyclohexane at 25oC [33].
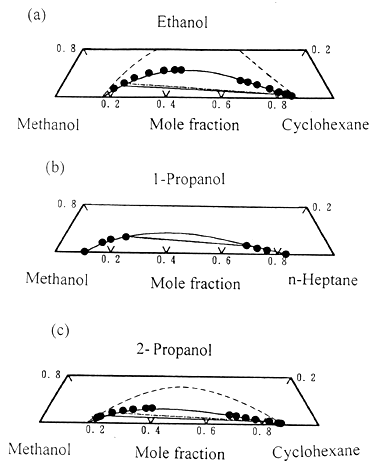
Fig.2 Liquid-liquid equilibria for the ternary systems consisting of two alcohol
mixtures; Experimental tie-line: ● - ●; Predicted lines: ——,
Extended UNIQUAC associated-solution model; - - - - - , UNIQUAC model.
(a). Methanol + ethanol + cyclohexane at 25oC [13];
(b). Methanol + 1-propanol + n-heptane at 25oC [34];(c).
Methanol + 2-propanol + cyclohexane at 25oC [23].
5. CONCLUSION
The single and multi-solvation forms of the extended UNIQUAC associated-solution model are
able to correlate well binary VLE and LLE and to predict ternary LLE for the mixtures
containing two associating molecules of alcohol, acetonitrile and aniline. The models
involving association and solvation constants fixed in this work are successful in the
accurate description of thermodynamic properties of the multicomponent mixtures. The
calculated results confirm fully good working abilities of the solvation models in
correlating binary VLE and LLE and in predicting ternary LLE of two associating molecules
by using binary parameters alone with association and solvation constants.
LIST OF SYMBOLS
aij |
binary
interaction parameters |
gE |
excess Gibbs
free energy |
hA |
molar
enthalpy of hydrogen-bond formation |
hAB |
enthalpies of
formation of chemical complexes AiBj |
KA
, KB |
association
equilibrium constants |
KAB |
solvation
equilibrium constant of formation of chemical complexes AiBj |
P |
total
pressure |
Pi0 |
saturated
vapor pressure of pure component i |

|
area
parameter of pure component i |
 |
modified area parameter of pure
component i |

|
volume
parameter of pure component i |
 |
modified volume parameter of
pure component i |
R |
universal gas
constant |
|
sums as defined by eqns. (24)
and (25) |
|
sums as defined by eqns. (26)
and (27) |
T |
absolute
temperature |
|
true molar
volume of the ternary mixture |
|
true molar
volume of pure component i |
xi |
liquid-phase
mole fraction of component i |
yi |
vapor-phase
mole fraction of component i |
Z |
lattice
coordination number |
Greek letters
gi |
activity
coefficient of component i |
qi |
surface
fraction of component i |
sP, sT, sx, sy, |
standard
deviations in pressure, temperature, liquid mole fraction and vapor mole fraction |
τij |
adjustable
binary parameters |
Fi |
segment
fraction of component i |
F ' i |
modified
segment fraction of component i |
F
'A1,F 'B1 |
monomer segment fractions of
associating components |
F '0C |
monomer
segment fraction of non-associating component |
i |
vapor phase fugacity coefficient
of component i |
i 0 |
vapor phase
fugacity coefficient of pure component i |
|
|
REFERENCES
[1] Tamura K, Chen Y, Yamada T. Fluid Phase Equilibria, 2001, 191: 15.
[2] Nagata I. Fluid Phase Equilibria, 1985, 19: 153.
[3] Brandani V. Fluid Phase Equilibria, 1983, 12: 87.
[4] Stokes R H, Burfitt C. J. Chem. Thermodyn., 1973, 5: 623.
[5] Nagata I, Ohtsubo K. Thermochim. Acta, 1986, 97: 37.
[6] Nagata I, Tamura K. Thermochim. Acta, 1988, 124: 53.
[7] Vera J H, Sayegh S G, Ratcliff G A. Fluid Phase Equilibria, 1977, 1:113.
[8] Spencer C F, Danner R P. J. Chem. Eng. Data, 1972, 17: 236.
[9] Hayden J G, O'Connell J P. Ind. Eng. Chem. Process
Des. Dev., 1975, 14: 209.
[10] Prausnitz J M, Anderson T F, Grens E A, et al. Computer Calculations for
Multicomponent Vapor-Liquid and Liquid-Liquid Equilibria, Prentice-Hall:Englewood Cliffs,
NJ, 1980.
[11] Nagata I. Thermochim. Acta, 1987, 112: 187.
[12] Sugi H, Katayama T. J. Chem. Eng. Japan, 1978, 11: 167.
[13] Nagata I, Ohta T. J. Chem. Eng. Data, 1983, 28: 256.
[14] Nagata I. Thermochim. Acta, 1987, 114: 227.
[15] Nagata I. Thermochim. Acta, 1987, 119: 357.
[16] Nagata I, Katoh K. Thermochim. Acta, 1980, 39: 45.
[17] Maher P J, Smith B D. J. Chem. Eng. Data, 1979, 24: 16.
[18] Nagata. Thermochim. Acta, 1991, 186: 123.
[19] Varteressian K A, Fenske M R. Ind. Eng. Chem., 1937, 29: 270.
[20] Berro C, Péneloux A. J. Chem. Eng. Data, 1984, 29: 206.
[21] Iguchi A. Chem. Soc., 1978, 20: 66.
[22] Raticliff G R. Can. J. Chem. Eng., 1976, 54: 326.
[23] Nagata I. Fluid Phase Equilibria, 1984, 18: 83.
[24] Sfrensen J M, Arlt W.
Liquid-Liquid Equilibrium Data Collection,Binary Sysytems, Vol. V, Part 1, DECHEMA Chem.
Data Ser. DECHEMA,Frankfurt, 1979)
[25] Hwang S C, Robinson R L. J. Chem. Eng. Data, 1977, 22: 319.
[26] Sipowska J, Wieczorek S. J. Chem. Thermodyn., 1980, 12: 459.
[27] Nagata I, Ohta T, Uchiyama Y. J. Chem. Eng. Data, 1973, 18: 54.
[28] Berro C, Neau E. Fluid Phase Equilibria, 1981, 7: 41.
[29] Kharin S E, Perelygin V M, Smirnov V S. Izv. Vyssh. Ucheb. Zaved. Khim Technol.,
1969, 12: 1695.
[30] Berro C. International Data Series, 1979, 2: 90.
[31] Freshwater D C, Pike K A. J. Chem. Eng. Data, 1967, 12: 179.
[32] Nagata I. Thermochim. Acta, 1988, 127: 109.
[33] Nagata I. Thermochim. Acta, 1991, 186: 123.
[34] Nagata I. Thermochim. Acta, 1988, 127: 337.
|