Preparation of highly ordered mesoporous
silica materials and application as immobilized enzyme supports in organic solvent
Li B., Inagaki S, Miyazaki C,
Takahashi H
(Toyota Central R&D Labs., Inc., 41-1, Yokomichi, Nagakute-cho, Aichi 480-1192, Japan)
Received Jul. 24, 2000
Abstract The hexagonal mesoporous silica
materials FSM-16, MCM-41 and SBA-15 having a highly arrange in order of the 2-dimensional
structure were synthesized by using different silicon sources and surfactants. Their pore
size can be uniformly controlled by the combined use of the surfactants having different
alkyl chains length and swelling agents (triisopropyl benzene). The pore-diameter of
FSM-16 and MCM-41 can be expanded to 100Å, SBA-15 is 150Å. In the FSM-16 derived from
Kanemite (silicon source) and MCM-41 from sodium silicate, its anionic characteristics on
the pore-wall may be higher than SBA-15 derived from oligomeric tetramethoxysilane (TMOS)
is also reported. A enzyme, horseradish peroxidase (HRP), was adsorbed in the manner of
single immersion method on the silica mesoporous materials, FSM-16, MCM-41 and SBA-15 with
various pore diameters from 30 - 90Å and the level of adsorption of enzymes and their
enzymatic activity in an organic solvent were studied. FSM-16 and MCM-41 showed larger
amount of adsorption of HRP than SBA-15 or silica gel, when the pore sizes were larger
than the 50Å. The increased enzyme adsorption capacity may be due to the surface
characteristics of FSM-16 and MCM-41. The mechanism of enzyme adsorption in mesopore was
suggested to be the ionic interactions. The anionic surface character and size fitting
between pore sizes and the molecular diameter of HRP was important to realize the high
enzymatic activity in organic solvent.
Keywords mesoporous silica, surfactant, swelling agent, enzyme, activity.
1 INTRODUCTION
The technique for environment protection has become increasingly important. The clear
bio-decompositive methods have been noted in recent years, and peroxidases (heme enzyme)
have been widely utilized in some applications such as depolymelization lignin or coal by
Ryoo et al [1, 2], and then used for decomposition of dioxin was also proposed [3].
However, this native enzyme can be not directly used to organic solvent, because of
inactivation. In order to achieve a homogeneous system and efficient reactivity, enzyme
should be modified by chemical reagent [4-9] or be immobilized onto the some
supports [10] and keeping activity in organic solvent. The fixation of
biologically active molecules onto inorganic materials combines the high selective
activity of the active molecule such as enzymes or antibodies with the chemical and
mechanical stability of the support. The combination is very useful for the field of
biocatalysis and chemical sensing [11]. MCM-41 (40Å) type mesoporous materials
have been used as supports of small globular enzymes, such as cytochrome C (Mr=12.3K.,
average molecular diameter, about 30Å) had been reported by Balkus Jr et al. [10,
11]. Since the pore diameter of the used MCM-41 is lower than 34Å, relatively large
molecular enzymes, such as horseradish peroxidase (HRP, Mr>44K, average molecular
diameter, about 48Å) not immobilized in the mesopore.
A molecular HRP is incorporated into cavity of porous solid has become
possible in recently, due to the perfection of the technique synthesized uniformly large
pore mesoporous materials [7]. Mesoporous referred to as FSM-16, MCM-41 and
SBA-15 etc. siliceous materials are extremely noted. Since mesoporous materials MCM-41
(hexagonal type), and 48 (cubic type) families were discovered by Mobil Corporation
researchers [12,13] in 1992, recently, there has been a considerable
development to research mesoporous silica materials about its physical properties [14-16],
swelling pore size [17, 18] and other application [19]. Bagshaw 20)
and Stucky group et al. [21-24] successfully synthesized large pore-diameter
(40 - 300Å) silicate that SBA-15 is called. There are mesoporous silica that have highly
ordered 2-dimensional hexagonal structure (p6mm) by using nonionic poly (alkylene oxide)
triblock copolymers (PEO-PPO-PEO) and tetramethyl orthosilicate (TMOS) as silica source in
acidic media, whole different way as MCM-41. As is well know, MCM-41 periodic mesoporous
silicate was prepared in autoclave using cationic cetyltrimethylammonium (CTMA+ )
surfactants and water-glass or TMOS as silica source in alkali media for 5-7 day. After
calcination it yield a hexagonal ordered porous solid with uniform pore-diameter of 15 to
46Å. Their pore diameters can be extended to 100Å in presence of TMB [13].
Yanagisawa et al. [25, 26] earlier suggested a simple way of synthesized
silica-based mesoporous materials in comparison with MCM-41, namely prepared from a
layered polysilicate (Kanemite) in atmosphere for about 10 hours. The obtained mesoporous
solid is called as FSM-16 (Folded Sheets Mesoporous material) by Inagaki et al. [27-29].
FSM-16 also is a regular array of uniform 2-d hexagonal mesoporous, which pore-diameter
can be tailored from 15 to 50Å by addition of a swelling agent such as TMB. Chen et al.
[28] confirmed that their thermal and hydrothermal are higher than MCM-41. During
process of forming ordered silicate crystal structure for FSM-16 and/or MCM-41, template
forming porous skeleton was found accomplished through cationic exchange between Na+
ions on inter layer of kanemite and cationic surfactant. While in the case of SBA-15,
condensation of polysilicate was promoted by formed hydrogen bond between silanol groups
of TMOS and alkoxyl groups of triblocks oligomeric surfactant. The surface electrification
in the FSM-16 and MCM-41 would be different from SBA-15. Therefore, we considered
utilizing this electrics difference to immobilization for action protein.
In this study, we showed that the surface characteristics of the
mesoporous silica, and matching of the sizes of the enzyme molecules and the pore
diameters of the mesoporous silica are essential for the stabilization of enzymes.
2. EXPERIMENTAL
2.1 Materials
All chemicals were commercially available from TCI (Tokyo Kasei Kogyo Co., LTD), KANTO
(Kanto Chemical Co., INC.), and WAKO (Wako Pure Chemical Industries, Ltd.). Surfactants
were obtained from LION or BASF, were used without further purification, including the
following: CTMABr, cetyltrimethylammonium bromide (TCI); STMACl, stearyltrimethylammonium
chloride (TCI); DCTMACl, docosyltrimethylammonium chloride (LION); P104, (Mw
4948), HO (CH2CH2O)18 (CH2CH2CH2O)58(CH2CH2O)18H
(BASF).TMOS, tetramethyl ortho silicate; Kanemite (ideal
formular: NaHSi2O5.3H2O), was
obtained from Nippon Kagaku Kogyo corp. Horseradish Peroxidase (HRP) was obtained from
Calzyme Laboratories, Inc. U.S.A.. Oxidizing agent, tert-butyl hydroperoxide (solution 80%
in di-tert-butylperoxide) was obtained from MERCK.
2.2 Syntheses of mesoporous silica materials
Mesoporous silica FSM-16 materials was synthesized at 80°C using CTMABr, STMACl or DCTMCl
as the templates, and kanemite as the silicon sources [28]. A typical
preparation, 10 g of kanemite was dispersed in water with stirring at the room temperature
for 1 h, and then it is filtrated. The filter layer was dispersed in 200 ml of aqueous
solution of CTMABr (0.1 M), and the mixture was heated at 80°C for 2 h under stirring.
Then the pH of the suspension was adjusted to 8-9 by adding 2 N HCl aqueous
solution, and heated at 80°C for 2 h under stirring. The resulted products was filtered,
washed with deionized water repeatedly, and air-dried at RT to get an as-synthesized of
FSM-16 materials. The calcination was carried out by slowly increasing temperature from
room temperature to 550°C in air for 2 h and heating at 550°C for 6 h to remove
the organic fraction. 1, 3, 5-trimethylbenzene (TMB) or1, 3, 5-triisopropylbenzene (TIPB)
was used as the swelling agent for the syntheses of FSM-16 with larger pore sizes. In such
as the case, the reactant was prepared following procedure. Above filter layer (kanemite)
was dispersed in water of 100 ml, then this supernatant mixed with 100 ml of 0.2M CTMABr
containing 0.06 mol TIPB, or 100 ml of 0.2M DCTMACl containing 0.08 mol TIPB. The set of
operating conditions were the same to that described above. The MCM-41 materials were
synthesized by using water glass as the silicon sources according to method of Beak et al.
[13]. SBA-15 materials were synthesized at 35-80°C using a triblock copolymer
type nonionic surfactant P104 as the structure-directing agent and tetramethyl
orthosilicate (TMOS) as a silica source by the method of Stucky et al. [21-24].
In a typical preparation, 6.0 g of P104 was dissolved in 200 ml of deionized
water and then added 20 g 12N HCl with stirring at 45°C, and that 1-10 ml TIPB was added
into that solution for 10 min with stirring, then 10.5 g TMOS was added into that the
solution for 10 h with stirring at 45°C. The mixtures were aged at 45°C overnight. The
next following, the mixture was hydrothermally treated at 80°C for 10 h with refluxing.
Following treatments of the resulted products were the same to that described above.
2.3 Immobilization of enzyme
An Immobilized enzyme in supports was prepared following procedure. 0.25 g of
FSM-16 or SBA-15 powder was added to 5 ml of a 10 mg/ml an aqueous solution (10 mM
phosphate buffer, pH7.0) of HRP in centrifuge tube. The mixture was stirred at 4°C for 16
h and centrifuge at 20,000×g for 10 min at 4°C, and then the pellet was washed with
deionized water three times and air-dried.
2.4 Measurement of enzymatic activity
For evaluation of the catalytic activity of the HRP immobilized in mesoporous silica,
the oxidative reaction of 1, 2-diaminobenzene in toluene was selected
tert-butyl-hydroperoxide being used as the oxidant. A portion 20 ml of 50 mM 1,
2-diaminobenzene in anhydrous toluene and 5 ml of 1.1 M tert-butylhydroperoxide in decane
were mixed. The reaction was initiated by adding a complex of mesoporous solid immobilized
HRP containing 1 mg protein with constant stirring at 37°C. The conversion at 37°C in 7h
via the oxidative reaction was determined spectroscopically by measuring the
absorbance of the supernatant at 470 nm.
2.5 Analyses
The structures of the above products were confirmed by means of X-ray powder diffraction
(XRD) using a Rigaku RINT-2000 or RAD-B diffractometer equipped with Cu Ka radiation source [28]. The
nitrogen adsorption and desorption isotherms at 77 K were measured using a QUANTACHROME
Autosorb1 system. The sample was pretreated at 150°C 2 h in the outgasser (vacuum line).
Specific surface areas were calculated by the BET method using the adsorption data at the
range from P/P0=0.05 to 0.35 just below the capillary condensation, and the
pore diameter distribution curve was derived from the adsorption branch by the BJH method.
The pore volume was taken as the P/P0 where the isotherm sharply increased.
Amounts of enzyme immobilized on to the supports were spectroscpically determined by
calculating the difference of absorbency of the supernatant at 403 nm for HRP. The
conversion of organic oxidative reaction was determined at 470 nm. JASCO Ubest-55 UV/Vis
spectrophotometer was used for analysis. The physicochemical parameters of FSM-16/d, MCM-41/d
and SBA-15/d (d, pore diameter) materials are summarized in Table 1. Silica
gel was purchased from FUJI SILYSIA CHEMICAL LTD.
Table 1 The characteriztion of synthesized mesoporous silica matericals
Pore characteriztion value |
FSM-16 |
FSM-16 |
FSM-16 |
MCM-16 |
MCM-41 |
MCM-41 |
MCM-41 |
SBA-15 |
SBA-15 |
SBA-15 |
/3.0* |
/5.0 |
/7.0 |
/9.0 |
/3.0 |
/5.0 |
/7.0 |
/5.0 |
/7.0 |
/140 |
Pore-diameter**, j/nm |
3.1 |
5.1 |
6.9 |
8.9 |
3.0 |
4.9 |
6.7 |
5.0 |
6.8 |
13.8 |
Total pore-volume/cm3g-1 |
0.84 |
0.95 |
1.22 |
1.63 |
0.88 |
1.39 |
1.42 |
0.56 |
0.78 |
1.04 |
BET Surface area/m2g-1 |
964 |
848 |
733 |
770 |
797 |
749 |
720 |
695 |
759 |
836 |
Unit cell parameter***, a0/nm |
4.7 |
7.3 |
9.4 |
11.7 |
4.7 |
6.8 |
8.3 |
10.1 |
11.4 |
16.9 |
Wall thickness/nm |
1.6 |
2.3 |
2.5 |
2.8 |
1.7 |
2.1 |
1.6 |
5.1 |
4.65 |
3.1 |
*) The whole number of approximate with
messuring value for pore-diameter; **) The top value of peak on pore-diameter distribution
curve; ***) X ray diffraction patterns, a0
3. RESULTS AND DISCUSSION
3.1 Designing mesopore
In order to immobilize HRP (approximate diameters ca. 48Å) in the mesopores, we
synthesized various mesoporous silica materials, such as FSM-16, MCM-41 and SBA-15, having
large pore diameters (more than 50Å). The pore size of mesoporous silica materials can be
increased by the addition of swelling agents such as TMB, TIPB or other organic compounds.
It was known that the arranged order of the pore structure decreases if the TMB/surfactant
ratio exceeds 1.0. We have successfully synthesized highly ordered large pore mesoporous
silica materials using TIPB even with a TIPB/surfactant ratio of up to 3.0. The pore
diameter was calculated with the adsorption isotherm of nitrogen. The power X-ray
diffraction patterns of mesoporous silica materials are shown in Fig. 1.
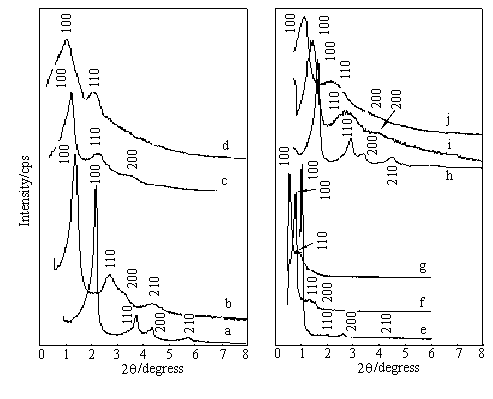
Fig. 1 Powder X-ray diffraction patterns of calcined mesoporous silica materials.
Spectra were also recorded with a Rigaku RINT-2200 diffractometer. (a) FSM-16/30, (b)
FSM-16/50, (c) FSM-16/70, (d) FSM-16/90, (e) SBA-15/50, (f) SBA-15/90, (g) SBA-15/140, (h)
MCM-41/30, (i) MCM-41/50, (j) MCM-41/70
The pattern of FSM-16/30 (a) or 50 (b),
MCM-41/30 (h) or 50 (i), and SBA-15/50 (e) or 92 (f) is typical of a well-ordered
specimen, and shows an intense (100) diffraction peak and three higher ordered hexagonal
structure (110), (200) and (210) peaks. However, porous crystal regularities decrease with
increasing pore sizes, i.e. the patterns of FSM-16/70 (c) and MCM-41/70 (j) show a highly
intense (100) peak, and weak (110) and (200) peaks, but a (210) peak was not observed. The
patterns of FSM-16/90 (d) and SBA-15/140 (g) only show a highly intense (100) peak and a
weak (110) peak, other peaks not being observed. Table 1 lists the tentative pore
diameters (distribution curve top), specific surface areas and total pore volumes of the
calcined porous silica materials. Increasing addition of TIPB increased unit cell
dimension, tentative pore diameter, and pore-volume. The specific surface areas of FSM-16
decreased with the increasing pore diameter, and wall thickness was increment. However, in
the case of SBA-15 is that surface area was a little increment and that wall thickness was
decrement. Mesoporous silica materials having a highly arrange ordered were prepared at
that the ratio of TIPB to surfactants were lower than 3.0 for FSM-16 and MCM-41, and 1.0
for SBA-15.
3.2 Immobilized peroxidase and the enzymatic activity
Enzyme molecules can be fixed in the pores of MCM-41 by simply immersing the mesoporous
material in the enzyme solution [10, 11]. To clarify the effects of the surface
characteristics of mesoporous materials on the adsorbed amounts of enzymes, we
investigated two types of mesoporous silica materials. One was prepared using a cationic
surfactant (MCM-41 and FSM-16) and the other using a non-ionic poly (alkylene oxide)
triblock copolymers (SBA-15). Fig. 2 shown adsorptive amounts of HRP for various
mesoporous materials. For FSM-16 or MCM-41, whose pore diameter is over 50Å, the loaded
amounts of enzymes reached values exceeding 80 mg per 1.0 g of support. When the pore
diameters are far larger than those of enzymes, such as in FSM-16/90, the loaded amounts
reached over 180 mg/g support. In contrast, FSM-16/30, whose pore diameter is far smaller
than those of enzyme molecules, the loaded amounts of enzymes were lower than 35mg /g
support. This result means that all enzymes only adsorbed on outer surface of FSM-16/30.
In the case of MCM-41, the adsorbed amounts were a little smaller than in that of FSM-16.
The enzyme loading in a silica gel with an extensive pore distribution was about 50 mg/g
support (data not shown). However, the enzyme loading in SBA-15 materials was lower than
30mg/g support. These values were far less than those for FSM-16 or MCM-41. This may be
due to the formation of electroneutralities in wall surface, made from using nonionic
surfactants. The adsorptive abilities was the order as FSM-16>MCM-41>>SBA-15 in
various mesoporous materials. This result possibly suggested that existing ionic
interaction between silanol groups (-SiOH) of surface of mesopore and surface amino acid
groups (NH+) of enzyme.
If ideally calculate total length (L) of 2-d hexagonal pore channel by
using its surface area, and assuming that per enzyme molecule closely arrange in channel,
then following formula (1) and (2), 1 g of FSM-16/90 can hold ca. 2.816×1019
HRP molecules by calculating. Namely 183 mg (ca. 2.505×1018 molecular) HRP of
largest value occupied 8.9% of space.
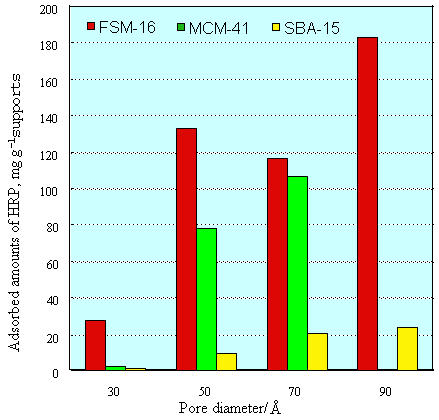
Fig. 2 Adsorption of enzyme (HRP) in various porous silica with various different
pore-diameter at 4°C for 20 h, HRP, 10 ml deionied water solution of 10 mg/ml.
L= s/2rp
(1)
N= 4v/3rez3
(2)
L: length of channel, nm; S: surface area of FSM-16/90, m2/g;
rp: radius of pore, nm;
V: total pore volume, cm3/g; N: numbers of enzyme;
rez: spherical molecular radius of enzyme.
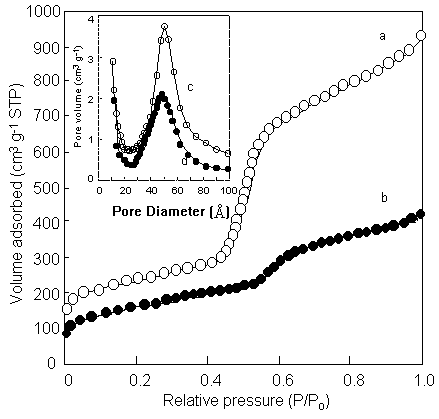
Fig. 3 Changes of N2 adsorption isotherms and the pore-diameter distribution curve
before (a) and after (b) loading HRP in FSM-16/50 .
The adsorption isotherms
of nitrogen and pore distribution curves for the FSM-16/50 material before and after HRP
loading are shown in Figure 3. The pore volume of FSM-16/50 decreased after the
immobilization treatment. The pore volumes of the FSM-16/50 materials after enzyme loading
were ca. 45.2 % of that of the FSM-16/50 material before enzyme loading. The pore volume
of FSM-16/30 was the same before and after enzyme loading. This indicates that enzyme
molecules do not occupy the mesopore space of FSM-16/30 but exist on the outer surface of
particles. According to the XRD measurements, the structure of FSM-16 was maintained after
immobilization of the enzyme molecules. These results suggested that HRP molecules were
adsorbed in the pore spaces of that diameters is over 50Å. Enzyme immobilization onto the
silica mesopore is based on some interactions between the surface of pore wall and the
amino acid residues of the protein. These interactions include ionic bonds and
electrostatic forces between negative charge sites in silica pore-wall and positively
charged amino acid residues of the enzyme, or hydrogen-bond interactions with hydrophobic
residues, them can promote adsorption of enzyme onto the intrapore and stabilize the
enzymes.
In particular, horseradish peroxidase (HRP) modified surface groups
have been widely applied as an effective biocatalyst. Such as the organic synthesis [30],
HRP-catalyzed reaction is usually conducted in a mixture of a hydrophilic organic solvent
and an aqueous buffer solution for reaction. As increase the ratio of a hydrophilic
organic solvent, often cause the inactivation of enzymes [31-33]. Therefore,
the heterogeneity in the reaction might decrease enzymatic activity. If protect residual
groups of surface of with through modification or forming complex, then use of enzyme in
an anhydrous organic solvent is possible. Following, enzymatic activity in organic solvent
was investigated by using fixation of immobilized HRP. The enzymatic activities on the
oxidative reaction of 1, 2-diaminobenzene in an organic solvent with native HRP [9],
and immobilized HRP in FSM-16, MCM-41 and SBA-15 with various pore sizes are compared in
Fig. 4. The enzymatic activity immobilized HRP in FSM-16 and MCM-41 was high. In the case
of SBA-15, because of amounts of combined HRP is far less than FSM-16 or MCM-41, lower
activity is predictable. The original FSM-16 also exhibited a little activity (conversion,
ca. 20% for 7 h), due to existing acid sites in the silica framework of FSM-16. In
the case of only HRP had almost no activity in toluene. The conversion by FSM-16/50
immobilized HRP reached more than 75% (back value of activity of by mesoporous materials
are subtracted from calculation) after 7h. However, the conversion by FSM-16/30
immobilized HRP was low. Among other silica supports, when the pore diameter matched the
molecular size of HRP, the immobilized HRP in MCM-41/50 also showed high catalytic
activity, i.e. 63% after 7h. These results suggested that HRP immobilized in FSM-16/51 or
MCM-41/50 would form a stable complex. Image figure with simulation homology modeling of
peptide and protein that HRP immobilized in pore of mesoporous silica is shown in Fig. 5.
This stable incorporation can effectively resist enzymatic inactivation from the organic
solvent and drawn from inside of pore by stirring.
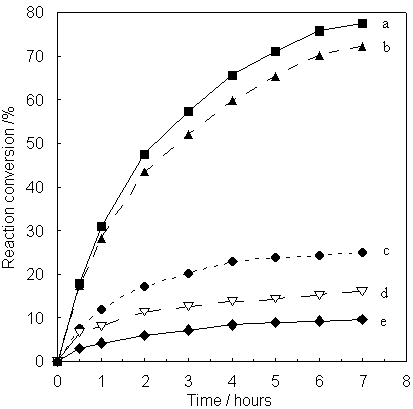
Fig. 4 Catalytic activity of HRP immobilized in various porous silica with various
pore sizes in organic solvent (toluene), (a) HRP-FSM16/50 , (b) HRP-MCM-41/50, (c)
SBA-15/50, (d) Original FSM-16/50, (e) native HRP, respectively.
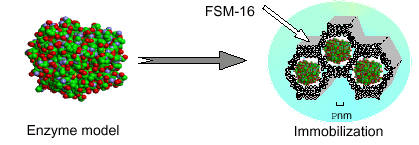
Fig. 5 Image models of immobilized HRP in FSM-16 with
pore-diameter 50Å using a computer schematic model. The FSM-16 model was constructed in
accordance with the folded sheet formation mechanism. The diameter of spherical molecular
HRP was about 46Å.
4. CONCLUSION
Mesoporous silica materials FSM-16, MCM-41 or SBA-15 having large pore sizes and highly
ordered. The 2-dimensional ordered hexagonal arrangements may be keep until pore-diameters
is expanded about 100Å for FSM-16 and MCM-41 or 140Å for SBA-15. Their pore wall surface
indicated different ionic characteristics. FSM-16 or MCM-41 materials showed the
adsorption of greater amounts of enzymes than SBA-15 materials. After treatment under
different thermal conditions or in an organic solvent, the residual activities of enzymes
immobilized in FSM-16 or MCM-41 materials were much higher than those in SBA-15/50
materials. These findings suggest that the surface characteristics of mesoporous silica
materials are critical for the adsorption and stabilization of enzyme molecules. When the
average mesopore sizes of FSM-16 just matched the molecular diameters of enzymes, the
immobilized enzymes exhibited the best stability. FSM-16 or MCM-41 materials with a
suitable mesopore size would be useful and applicable as bio-catalysts.
REFERENCE
[1] Ryoo R, Jun S. J. Phys. Chem. B., 1997, (101): 317.
[2] Corma A, Kan Q-B, Navarro M T et al. Chem. Mater., 1997, (9): 2123.
[3] Blinkorsky A M, McEldoon J P, Dordick J S et al. Appl. Biochem. Biotechnol., 1994,
(49): 153.
[4] Takahashi K, Kodera Y, Inada Y et al. Biochem. Biophy. Commun., 1985, (131): 532.
[5] Matsushima A, Kodera Y, Takahashi K et al. Biotechnol. Lett., 1986, (8): 73.
[6] Inada Y, Yoshimoto T, Matsushima T et al. TIBTECH, 1986, (3): 68-73.
[7] Takahashi K, Matsushima A, Inada Y et al. Biochem. Biophys. Res. Commun., 1986, (138):
283.
[8] Urrutigoity M, Souppe T. Biocatalysis, 1989, (2): 145.
[9] Okahata Y, Okazaki S, Goto M. Bull. Chem. Soc. Jpn., 1992, (65): 2411.
[10] Gimon-Kinsed M E, Jimenez V L, Balkus K J et al. Mesoporous Molecular Sieves, 1998,
(117): 373.
[11] Diaz J F, Balkus K J. J. Mol. Catal. B: Enzymatic, 1996, (2): 115.
[12] Kreseg C T, Leonowicz M E, Beck J S et al. Nature, 1992, (359): 710.
[13] Beck J S, Vartuli J C, Roth W T et al. J. Am. Chem. Soc., 1992, (114): 10834.
[14] Zhu H Y, Zhao X S, Do D D et al. Langmuir, 1996, (12): 6513.
[15] Nguyen C, Sonwane C G, Do D D et al. Langmuir, 1998, (14): 4950.
[16] Mokaya R, Zhou W Z, Jones W. J. Chem. Soc., Chem. Commun., 1999, 51.
[17] Sayari A, Liu P, Kruk M, Jaroniec M. Chem.Mater., 1997, (9): 2499.
[18] Namba S, Mochizuki A, Kito M. Mesoporous Molecular Sieves., 1998, (117): 257.
[19] Reddy K M, Wei B, Song C S. Catalysis Today, 1998, (43): 261.
[20] Bayshow S A, Prouzet E, Pinnavaia T J. Science, 1995, (269): 1242.
[21] Zhao, D-Y.; Feng, J-L.; Stucky, G. D; et al. Science, 1998, (279): 548.
[22] Yang P, Zhao D Y, Stucky G D et al. Nature, 1998, (396): 152.
[23] Yang P, Zhao D Y, Stucky G D et al. Chem. Mater., 1998, (10): 2033.
[24] Zhao D Y, Huo Q S, Stucky G D et al. J. Am. Chem. Soc., 1998, (120): 6024.
[25] Yanagisawa T, Shimizu T, Kuroda K et al. Bull. Chem. Soc. Jpn. 1990, (63): 988.
[26] Yanagisawa T, Shimizu T, Kuroda K et al. Bull. Chem. Soc. Jpn. 1990, (63): 1535.
[27] Inagaki S, Fukushima Y, Kuroda K. J. Chem. Soc., Chem. Commun., 1993, 680.
[28] Inagaki S, Fukushima Y, Kuroda K et al. Bull. Chem. Soc. Jpn., 1996, (69):
1449.
[29] Inagaki S, Fukushima Y, Kuroda K et al. Proceedings from the ninth
international zeolite conference (Ed by Ballmoos R V, Higgins J B, Treacy M M).
London: Butter Worth-Heinemann, 1992, Vol. I: 305-311.
[30] Okahata Y, Lim H J, Hachiga S et al. J. Membr. Sci., 1984, (19): 237.
[31] Okahata Y, Seki T. J. Am. Chem. Soc., 1984, (106): 8065.
[32] Takahashi K, Ajima A, Yoshimoto T et al. J. Org. Chem., 1985, (50): 3414.
[33] Kamiya N, Okazaki S, Goto M. Techniques., 1997, (11): 375.
|