Determination of ultra trace
amounts of zinc in biological materials by ICP-AES after preconcentration by an on-line
continuous precipitation-dissolution flow system
Fan Zhefeng, Du Liming,
Jin Xiaotao
(Center of Analysis and Test; Shanxi Normal University, Linfen 041004, China.)
Received May. 15, 2001; Supported by the Natural Science Foundation of Shanxi
(No.20011016).
Abstract A method for the determination
of zinc by inductively coupled plasma atomic emission spectrometry (ICP-AES) has been
developed. The method is based on the continuous precipitation of zinc as an ion pair
between tetra thiocyanate zinc and methyl violet, and then dissoluing the precipitate by
ethanol. The metal can be preconncentrated 40-fold using 10ml of sample solution by a
time-based technique at a sampling flow rate of 2.0 ml min-1. The linear range
from 0.1 to 6.5 mg g-1,
and RSD <4.0% could be expected. The detection limit based on 3s of the blank signal is 0.22 mg L-1 for
10ml of sample digest (0.022 mg g-1). The method demonstrates a high tolerance to
interference and the data obtained are in agreement with the certified value of a selected
reference material. This procedure was applied to the determination of zinc in some
biological materials.
Keywords Precipitation-dissolution; ICP-AES; zinc; biological materials.
1 INTRODUCTION
For the growth of both plant and animal life, the presence of small
quantities of zinc is essential [1]. As a bioessential element, zinc
participates in many biochemical processes. Zinc exerts beneficial effects on
cardiocirculatory function and prevention of black foot disease [2]. Therefore,
sensitive, selective and rapid methods for the determination of zinc are in great demand.
Spectrophotometry [3], atomic absorption spectrometry [4] and
inductively coupled plasma atomic emission spectroscopy [5], have been widely
applied to the determination of zinc at trace level. But these methods are not selectively
sensitive determination for zinc. Because of the extremely low concentration of zinc in
biological samples, and the complexity of the sample matrix, a preliminary
preconcentration step or selective separation from interference is usually necessary
before its determination. Flow injection (FI) on-line preconcentration procedures offer
the possibility of determining lower concentrations and avoiding matrix effects by
effective separation of the analysis from interference matrix components[6]. In
this hand, flow systems based on ion-exchange[7-8], sorbent extraction[9-10],
and coprecipitation and precipitation[11-12] have been successfully applied to
the determination of trace metals, but zinc determined by in flow system method is not
proposed at present.
In this paper, continuous precipitation-dissolution is used in
conjunction with inductive coupled plasma atomic emission spectrometry (ICP-AES) for zinc
preconcentration and determination in biological samples. The method involves zinc
precipitation as an ion pair formed between tetra thiocyanate zinc, Zn(SCN)42-
and methyl violet. The precipitate is dissolved in ethanol. The method allows the
determination of zinc over the range 0.1-6.5 mg.g-1.
2 EXPERIMENTAL
2.1 Apparatus
Zinc concentration measurements were done by an all-argon sequential Thermo Jarrell Ash
Corporation Atom Scan 25 inductively coupled plasma atomic emission (ICP-AES) spectrometer
(USA), equipped with a Babington V-groove Teflon nebulizer. RF power 1350W, observation
height 15 mm, coolant gas flow rate 20 L min-1, plasma gas flow rate 0.6 L min-1,
carrier gas flow rate o.5 L min-1, Zn(II) 213.858 nm lines
were measured. pHS-2 acid meter (Shanghai Tianda Co.) was used.
The flow system comprised a HL-1
peristaltic pump (Shanghai Huxi Co.), Poly (tetrafluorethylene) (PTFE) membrane filter
(0.8 mm
i.d.). This precipitate collector was effective in retaining the precipitate and did not
produce excessive back pressure, PTFE tubes (0.5and0.8mm i.d.) were employed.
Samples were digested in a high pressure asher (HPA system from Kurner,
Rosenheim), which permits the simultaneous digestion of seven samples in vessels at
controlled temperature and pressure. Materials and vessels used for trace analysis were
kept in 10% nitric acid for at least 24 h and subsequently washed three times with ultra
pure water before use.
2.2 Reagents
A standard zinc solution of 1000mg ml-1was
prepared from Zn(II) nitrate in 0.5mol.L-1 nitric
acid. From this solution, other diluted standard solutions were prepared. A 4×10-3mol.L-1 methyl violet solution was
prepared by dissolving 1.5758g of methyl violet in water and then diluted to 1000ml in a
volumetric flask. A 2.0×10-3mol.L-1 ammonium thiocyanate solution was
prepared by dissolving 0.1424g of the salt in water and diluted to 1000ml in a volumetric
flask. Nitric acid for sample digestion and 25% ammonia to adjust the sample pH were used.
All reagents were analytical reagent grade. The certified reference material used was tea
leaves GBW08505, humane blood serum GBW09135 and mussel GBW08571 from the National
Research Center for CRM's of China. Ethanol ,Ultra pure water
(18.3MW.cm-1).
2.3 Sample digestion
Approximately 0.25g of samples was accurately weighted in a quartz vessel and 3 ml of
distilled nitric acid was added. The vessel was closed and inserted in the HPA system. The
digestion program was equipped with a fast temperature ramp to 250°C(1min),a 3.0 min ramp
from 250 to 290°C and 9.0 min at 290°C, The pressure inside the system was about 100 bar
during the process. The vessels were then opened and the sample pH was adjusted to 2.0
with 25% ammonia, and subsequently diluted to 25ml with ultra pure water in a calibrated
flask, analytical blanks were prepared in parallel in the same way as the samples.
2.4 Procedure
The continuous flow manifold used for zinc
preconcontration is depicted in Fig.1. The sample or standard solution (10ml) containing
between 1 and 65 mg.L-1 zinc(pH 2), a 2.0×10-3mol.L-1
ammonium thiocyanate solution and the precipitating reagent(4.0×10-3mol.L-1
methyl violet solution) was introduced into the flow system using a time-based
technique (pumping time 5 min). The sample and ammonium thiocyanate streams were mixed in
the reaction coil, where formation of the anionic complex tetra thiocyanate zinc takes
place. Then, the tetra thiocyanate zinc formed and the precipitating reagent stream were
mixed in the precipitation coil, i.e. where formation of the insoluble ion pair occurs.
The precipitate was retained on a filter. The reagent stream was sent to waste via
switching valve SV2, and ultra pure water was aspirated by nebulizer in order to flush it
after each measurement. Then, in the dissolution step, the switching valve SV1 was
actuated, so that the ethanol stream passed through the precipitate in order to dissolve
it and sweep the zinc to the ICP-AES, peak response proportional to the amount of zinc
present in the sample volume introduced was obtained. No precipitating washing was
required.
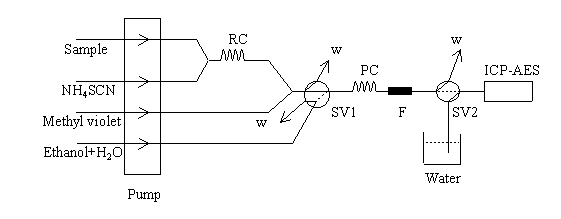
Fig.1 Schematic diagram of the
manifold used for on-line preconcentration of Zinc.
P. Peristaltic pump. RC. Reaction coil. PC. Precipitation coil.
SV. Switching valves. F. Filter. W. Waste lines.
ICP-AES. Inductive coupled plasma atomic emission Spectrometry.
3 Results and discussion
3.1 Optimization of reaction conditions
The influence of reaction conditions was studied by introducing 6ml (sample flow rate
2.0 ml min-1) of a standard solution containing 60 mg.L-1 zinc into the
system to establish the best reaction conditions for the precipitation reaction and
dissolution of the precipitate. Parameters were varied while keeping the FI variables
constant.
First, the complex reaction
between zinc and ammonium thiocyanate was studied. Ammonium thiocyanate forms complexes
with zinc including several coordination numbers, and this stoichiometry is function of
the [Zn2+]:[ SCN-1]ration. In order to optimum the ammonium
thiocyanate concentration to form a tetra thiocyanate zinc complex, the ammonium
thiocyanate concentration was studied between 1.0×10-4 and 8.0×10-3mol.L-1
introduced into the flow system at a flow rate of 0.5 ml min-1. When low
ammonium thiocyanate concentrations were used(<1.2×10-3mol.L-1) the
Zn(SCN)42- specie has not prevalent, and on the other hand, at high
concentrations (>6.0×10-3mol.L-1) complex with high coordination
numbers can be formed .Therefore an [SCN-] to [Zn2+] ratio near to 2×103
was chosen for subsequent use. The effect of pH on the formation of the complex and
subsequently on the precipitation of the ion pair was studied in the range0-6.0. The
intensity versus pH graph show a plateau from 2.0 to 5.0, below pH 1.5 and above pH 5.5 no
signal was obtained, A pH of 3.0 was selected for subsequent work. The influence of methyl
violet concentration was tested to obtain the best precipitation conditions of the ion
pair formed between Zn(SCN)42- and methyl violet. A methyl violet
concentration of 4.0×10-3mol.L-1 was found to be sufficient for
maximum response .The increase of methyl violet concentration did not affect the signal.
The influence of precipitating reagent pH to form the insoluble ion pair in the best
conditions was tested in the pH range 2.0-6.0. The maximum precipitation efficiency was
achieved at pH 3.0-5.0, and was constant throughout this range. So, a 4.0×10-3
mol.L-1 methyl violet solution pH of 4.0 obtained by diluting with ultra pure
water was selected. The effect of the dissolving reagent was investigated by using
ethanol. Dissolution of the precipitate was complete with ethanol and water (2+1). No
blank or precipitate washing was required because zinc concentration is very small and is
not detected directly without preconcentration.
3.2 Optimization of flow rate
The influence of the precipitation coil length was investigated between 50 and 500 cm
(0.5mm i.d.) at a constant flow rate, short reactor coils (less than 150cm) resulted in
short residence times of the plug. Hence mixing was insufficient, and the precipitation
reaction was in complete long reactors coil (above 300cm) increased the residence time of
the precipitate in the reactor, and caused it to be partly adsorbed on the inner walls of
the PTFE tubing. As result an increase dispersion of the filtrate plug and hence peak
width was obtained. A 200cm long precipitation coil was therefore chosen. The influence of
reaction coil length was investigated between 20 and 200cm. Maximum tetra thiocyanate zinc
complex was achieved at a length of 30cm.longer lengths up to 200 on gave rise to constant
signals. Short reactor coils (less than 30cm) resulted in short residence time. A reaction
coil of 30cm was therefore chose, sample flow rate was changed between 0.5 and 4.0 ml·min-1 at constant ammonium thiocyanate and
methyl violet flow rates of 0.5 ml·min-1 the analytical signal increased up to
2.5ml·min-1. Because the sample was less markedly diluted at the higher flow
rates and the residence time of the precipitate in the precipitation coil was shorter,
which entailed incomplete precipitation. The ammonium thiocyanate flow rate was studied
between 0.2 and 2.0ml min-1, intensity was constant up to 0.6ml·min-1,
a flow rate above 0.5 ml·min-1 entailed short residence time in the reaction
and precipitation coils. The methyl violet flow rate was tested between 0.2 and 3ml·min-1.
The detector response was constant up to 0.6ml min-1 at a flow rate above
0.6ml·min-1, a shorter residence time in the precipitation coil was achieved
and formation of insoluble ion pair was not complete. A sample flow rate of 2.0ml·min-1
a ammonium thiocyanate flow rate of 0.5ml·min-1, and a methyl violet flow rate
of 0.5 ml·min-1 were thus chosen as a compromise. The effect of the flow rate
of the dissolving regent was studied between 1.0 and 4.0ml·min-1. The flow
rate muse be higher than 2.0ml·min-1, because lower dissolving stream flow
rate resulted in broader lower peaks. The ethanol flow rate of 3.0 ml·min-1 was this selected.
3.3 Interference from co-existing ions
The effect of potential interference occurring in biological samples on the determination
of zinc was investigated using the optimized FI system. The metal ions individually were
added to a solution containing 20mg·L-1,
zinc was determined by ICP-AES. The results are shown in Table 1.
Table 1.Effect of ions
Interference ion |
Tolerance limit |
K(I), Na (I),Ca(II),
Mg( II) |
500mg |
Cu(II),Fe(III) ,Ni(II)
,Mn( II) |
50mg |
Pb(II) ,Cr (VI),Cr( III),Ti(IV),Mo(VI) |
20mg |
Cd(II),Hg(II),Co( II) |
5mg |
3.4 Calibration
The calibration graph obtained under the optimum conditions stated Fig.1 was linear over
the range 1-65 mg ·L-1 zinc for 10ml of sample (0.1-6.5 mg·g-1). The graph equation is I=1.4×10-4+4.8×10-3C;
r=0.998 (n=11) Where I is intensity and C the zinc concentration in mg·L-1. The preconcentration factor
calculated as the ration of the slopes of the calibration graphs obtained with and without
preconcentration (run by direct aspiration of zinc I=1.8×10-4+1.2×10-4C)
was 40. Sample volumes above than10ml can be used and this higher preancenrtation factor
can be achieved.
The precision of the continuous preconcentration method for the
determination of 2,4,8,10,20,50,60mg·L-1 zinc was in the range 1.2-3.3%(n=11). The detection limit calculated as
three time the standard deviation (s) of the blank (n=11) was 0.22 mg for 10ml of sample digest (0.022 mg·g-1).
3.5 Determination zinc in biological samples
The accuracy and applicability of the proposed method has been applied to the
determination of zinc in biological samples. The results are given in Table.2, which are
in good agreement with the certified values.
Table 2 Analysis of zinc in biological
samples
Sample |
Composition
|
Concentration
( mg·g-1)
|
Certified valve |
Found a |
Human blood
serum(GBW09135) |
K,0.178%±0.010; Na,3.12%±0.13; Mg,20.4±0.8; Cu,1.05±0.09; Ca,85.5±5.3; Fe,1.3±0.3;Pb(0.17) |
1.03±0.23 |
1.06±0.09 |
Tea leaves
(GBW08505) |
K,1.97%±0.13; Ca,0.284%±0.021; Mg,0.224±0.019; Na,142±13; Cu,16.2±1.9; Mn,766±28; Ni,7.61±0.48; Pb,1.06±0.10; Hg(0.004);
Cr (0.8); Ti (36) |
38.7±3.9 |
37.2±1.9 |
Mussel
(GBW08571) |
K,0.424%±0.010; Na,0.582%±0.007; Mg,0.197%±0.010; Ca,0.111%±0.002; Fe,221±7; Mn,10.2±0.9; Cu,7.7±0.5; Cd,4.5±0.3; Ni,1.03±0.07; Pb,1.96±0.05; Cr,0.57±0.04; Hg,0.067±0.004 |
138±5 |
137±2.1 |
- Average of five determinations ±standard
deviation.
4 CONCLUSIONS
Continuous preconcentration system described in this work was shown to be
an effective approach for improving the detection limits of ICP-AES for Zn determination.
This system is very selective showing high tolerance to interference from biological
samples matrix, and is fast, simple and suitable to determine zinc at trace level in the
samples with complex matrices.
REFERENCES
[1] Morgan S W X. Zinc and its Alloys and compounds, UK, Ellis Horwood,
Chichester, 1985, 70.
[2] Horng C J, Lin S R. Talanta, 1997, 45: 75.
[3] Kumar K, Indian. J.Che.Sect. A, 1996, 35A: 246.
[4] Mohamed K A, Kourashy E, Ghany A. Anal. Methods instrum., 1995, 2: 190.
[5] Wilharlitz P, Dreew S, Krismer R et al. Mikrochim, Acta., 1997, 125: 45.
[6] Fang Z, Flow Injection Separation and Preconcentration, VCH, Weinheim, 1993.
[7] Cespon-Pomero R M, Yebra-Biurrun M C, Bermejo-Barrera M P. Anal.Chim.Acta., 1996, 327:
37.
[8] Enriquez-Dominguez M F, Yebra-Biurrun M C, Bermejo-Barrera.M P. Analyst., 1998, 123:
105.
[9] Petit de Pena Y, Gallego M, Valcarcel M. J.Anal.At. Spectrom., 1994, 9: 691.
[10] Vanmol R M, Adams F. At.Spectrosc., 1996, 17: 176.
[11] Santelli R E, Gallego M, Valcarcel M. Anal.Chem., 1989, 61: 1427.
[12] Dittfurth C, Ballesteros E, Gallego M et al.
Spertrochim.Acta., 1996, 51B: 1935.
|