Preparation of Ti-HMS
mesoporous materials and their photocatalytic reactivity for the decomposition of NO into
N2 and O2
Zhang Jinlong, He Bin, Masakazu Anpo#
(Institute of Fine Chemicals, East China University of Science and Technology, Meilong
Road 130, Shanghai 200237, China; #Department of Applied Chemistry, Osaka
Prefecture University, Gakuen-cho, Sakai, Osaka 599-8531, Japan)
Received Nov. 26, 2002; Supported financially by the National Natural Science
Foundation of China (No.29476231), Foundation of Ministry of Education P.R.C., Shuguang
Plan of Commission of Education of Shanghai, and Nano-materials Special Item of Commission
of Shanghai Science and Technology.
Abstract Titanium oxide species
included within the framework of mesoporous zeolites (Ti-HMS) prepared at ambient
temperature were studied using nitrogen adsorption/desorption over a wide range of
relative pressures. It was shown that there are some differences in surface areas, pore
size, and widths of pore size distributions with the different titanium content
framework-substituted Ti-HMS. These mesoporous materials exhibited high and unique
photocatalytic reactivity for the direct decomposition of NO into N2, N2O
and O2 at 275K.
Keywords Titanium oxide, mesoporous material, photocatalyst,
photocatalytic decomposition
1 INTRODUCTION
NOx is an especially harmful atmospheric pollutant which
causes acid rain and photochemical smog. The removal of nitrogen oxides (NOx, x= 1, 2),
i.e., the direct decomposition of NOx into N2 and O2, has been a
great challenge for many researchers. [1-8] On the other hand, photocatalysis
is an attractive low-temperature, nonenergy-intensive approach for chemical waste
remediation. TiO2 has been extensively used in the field of heterogeneous
photooxidation catalysis for environmental cleanup because TiO2 is nontoxic,
stable, and inexpensive. Their band gap excitation can occur from the UV end of the solar
spectrum. Therefore, TiO2 is the most investigated photocatalytic system and
has been found to be capable of decomposing a wide variety of organics and inorganics in
both liquid phase and gas phase.[9, l0] Highly dispersed titanium oxides in
zeolite or silicalite cavities were used in the reduction of CO2 with H2O
to produce CH3OH and the direct decomposition of NO into N2
and O2.[6, 11] Both latter reactions are important with regard
to the greenhouse effect and global air pollution control and the development of
environmentally friendly technologies using solar energy.[2, 11, 12]
Especially, ordered mesoporous materials (OMMs) [13-16] have recently attracted
a lot of attention because of the presence of an array of uniform size pore channels
(hexagonal pores) having negligible pore-networking or pore blocking effect.[13]
Prominent features of these materials include the tunability of their pore diameter (in
the range of 1.5 - 10 nm) by suitable modification of the synthesis procedure, a high
surface area of 600-1300 m2/g, and ease of modification of the surface
properties by incorporating hetero-atoms such as Al, B, Ti, V, and Mo, which make
them suitable for a wide variety of applications in catalysis, environmental cleanup,
solid-phase extraction, and advanced materials design. [17-19] One of the most
important issues in the synthesis and application of OMMs is a reliable characterization
of their structural and surface properties. Gas adsorption (especially nitrogen
adsorption) is commonly used to study these novel materials. The isomorphous substitution
of Si by Ti into the zeolite framework provides useful catalysts for the oxidation of
organic compounds under mild conditions. Zeolites TS-1 and TS-2 show good activity and
selectivity in the oxidations of phenol to cathecol and hydroquinone, olefins to epoxides,
and alkanes to alcohols.[20] As described in ahead, the preparation of
inorganic mesoporous materials has attracted considerable attention because of their
possible use in catalysis and sorption, as well as in host-guest chemistry. However,
relatively little is known concerning the catalytic activities of titanium-substituted
mesoporous molecular sieves, especially for the Ti-HMS. The physicochemical and
reactivity/selectivity properties of oxide catalysts are often a strong function of their
structural characteristics. In those materials, Ti atoms are shown to substitute Si in the
silics framework or matrix to form tetrahedral TiO4 units, which function as
active and high selective for the formation of N2 in the decomposition
of NO. However, the structural characteristics of titanium oxide species included within
the framework of mesoporous zeolites (Ti-HMS), and their relation with the physicochemical
and reactivity/selectivity properties are poorly understood due to the lack of systematic
fundamental studies. Therefore, the present investigation focuses on the molecular
structure of the highly dispersed titanium species within the mesoporous materials which
are sensitive to the environmental conditions. Furthermore, photocatalytic decomposition
of NO as a probe reaction was employed to study the relationship between the reactivity
and selectivity of Ti included mesoporous photocatalyst and their local structures.
2 EXPERIMENTAL
In this paper, one method was successful in the synthesis of Ti-HMS described by Thomas J.
Pinnavaia research group.[21] A typical procedure for the synthesis is as
follows: Tetraethyl orthosilicate (TEOS), tetraisopropyl orthotitanate (TIPOT), and
long-chain alkylamine surfactant were used as a source of silica, titanium, and template,
respectively. Ti-HMS was synthesized via a neutral SoIo templating
pathway using dodecylamine (DDA) as the surfactant and ethanol (EtOH) as a co-solvent. In
a typical preparation the TEOS/TIPOT solution was added to the solution of DDA in water
and ethanol under vigorous stirring. The molar composition of the reaction mixture was x
Ti: 1.0 Si: 0.20 DDA: 9.0 EtOH: 160 H2O. The reaction mixture was aged at
ambient temperature under vigorous stirring for 24 h in order to obtain the crystalline
product. All the samples were filtered, washed thoroughly with water, dried at ambient
temperature, and calcined at 923 K for 4 h. The final catalysts were denoted as Ti-HMS(x).
The actual composition of the Ti-HMS photocatalysts was determined by atomic absorption.
Samples were dissolved in HF solutions and measured on a Perkin-Elmer system
with a sensitivity of 1.7 mg/ml.
The surface area, pore volume, and pore size distribution of samples
heated at 600 - 800 ºC were measured by a Quantachrome Corporatin
NOVA-1000 nitrogen gas sorption analyzer. All the samples were preheated at 250ºC under
vacuum in order to remove the adsorbed water remaining in pores.
TiO2 powered catalysts (P-25: anatase 92%, rutile 8%) were
supplied as a standard reference catalyst by the Catalysis Society of Japan. The
photocatalytic reactions of NO molecules were carried out with the catalysts (100 mg) in a
quartz cell with a flat bottom (60 ml) connected to a conventional vacuum system (10-6
Torr range). Prior to the photoreactions and spectroscopic measurements, the catalysts
were degassed at 725 K for 2 h, heated in O2 at the same temperature for
another 2 h, and finally evacuated at 475 K to 10-6 Torr. UV irradiation of the
catalysts in the presence of NO (7.8 mol) was carried out using a 75 W high-pressure Hg
lamp ( l> 280 nm ) at
275 K. The reaction products were analyzed by gas chromatography.
3 RESULTS AND DISCUSSIONS
The actual compositions and surface area of Ti-HMS are shown in Table 1. The actual TiO2
content of the Ti-HMS samples were analyzed by atomic absorption and the results
are slightly in variance with the expected value. Unless otherwise notified, all TiO2
content mentioned in the paper are referred to the nominal values.
Table 1. The actual
compositions, surface area, pore diameter, and pore volume of Ti-HMS(1 ), Ti-HMS(2) and
Ti-HMS(10).
Sample |
Actual Ti cotent (w%) |
surface area
(m2/g) |
pore
diameter
(Å) |
total pore volume
(cm3/g) |
Ti-HMS(1) |
1.26 |
871.43 |
29.79 |
1.2659 |
Ti-HMS(2) |
2.30 |
849.64 |
24.62 |
1.0654 |
Ti-HMS(10) |
11.6 |
745.86 |
24.45 |
0.9182 |
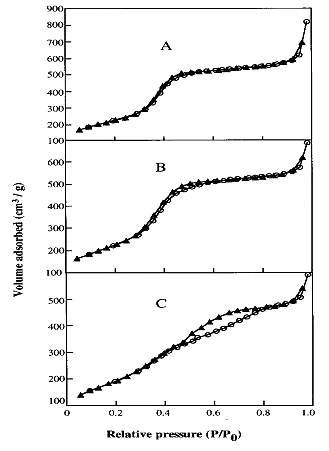
Figure 1. Nitrogen adsorption ( o ) and desorption (¡ø) for
Ti-HMS(l) (A), Ti-HMS(2) (B), and Ti-HMS(10) (C), respectively.
Figure 2. Changes of the pore size distribution of (A) Ti-HMS(1), (B)
Ti-HMS(2) and (C) Ti-HMS(10) with different Ti content.
Nitrogen adsorption
isotherms for Ti-HMS materials are shown in Figure 1, and selected structural properties
are listed in Table 1. From Figure 1, it can be known that Type IV isotherms are obtained
for these three mesoporous Ti-HMS materials.[22] The pore size distributions
(PSDs) obtained using the well-known Barrett-Joyner-Halenda (BJH) technique in the
mesopore range for the Ti-HMS samples are shown in Figure 2. As it can be seen in Figures
1 and 2, adsorption capacities as well as widths and positions of the pore size
distribution peaks for Ti-HMS materials under study exhibited appreciable
dependence on the Ti-content. Figure 1 shows that the pore filling (by capillary
condensation ) for Ti-HMS is restricted to a narrow range of P/P0 = 0.3 - 0.5,
which is a typical feature for mesoporous materials. The inflection point for Ti-HMS (10)
(Figure 1 C) is, however, less unambiguous as compared with that of Ti-HMS(1) and
Ti-HMS(2). Consequently, the pore size distribution of Ti-HMS(10) is much broader than
that of Ti-HMS(1). The results imply that the mesoporous structure of Ti-HMS(10) is
less perfectly arranged than that of Ti-HMS(1). In addition, according to the Table 1, the
surface area, the pore diameter and the total pore volume of those mesoporous materials
become decreasing with increasing Ti content. In Fig. 2, the PSDs gradually shifted
toward smaller pore sizes, and the peaks became lower as the content of Ti increased. In
agreement with a decreased intensity of its XRD peaks, Ti-HMS(2) and Ti-HMS(10) had
somewhat broader PSD than the Ti-HMS(1) sample, [23]
which indicates that large titanium content in the synthesis gel led to lowering of
structural uniformity of the resulting HMS material.
Combined in-situ UV-vis spectra, photoluminescence, and XAFS
spectroscopes¡¡illustrated that:[23] For low Ti content sample Ti-HMS(l), the surface Ti
atoms predominantly located in isolated Ti-O sites, existing in tetrahedral coordination,
while with increasing Ti content, there were more octahedral coordinated titanium
oxide species in those photocatalysts. ¡¡In the
samples of Ti-HMS(10), the titanium oxide species did not exist in the framework of HMS,
existing in the mixture of tetrahedral, pentahedral and octahedral coordination.
UV irradiation of powdered TiO2 and Ti-HMS
photocatalysts in the presence of NO was found to lead to the evolution of N2,
O2, and N2O in the gas phase at 275 K with different yields
and different product selectivities. The yields of these photo-formed N2, O2,
and N2O increased linearly to the UV irradiation time and the reaction
immediately stopped when irradiation was ceased. The formation of these reaction products
was not detected in the dark conditions nor in UV irradiation of HMS itself without
titanium.
The photocatalytic reactivities of various titanium oxide catalysts for
the direct decomposition of NO are shown in Figure 3. Of special interest is the
comparison of the photocatalytic reactivities of Ti-HMS catalysts with that of the widely
used bulk TiO2 powdered catalyst. It can be seen that the specific
photocatalytic reactivities of the Ti-HMS catalysts, which have been normalized for the
unit amount of TiO2 in the catalysts, are much higher than the bulk TiO2
catalyst.
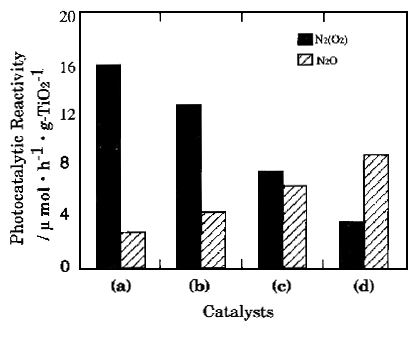
Figure 3. Products
distribution of the photocatalytic decomposition of NO on the different photocatalysts:
(a) Ti-HMS(l); (b) Ti-HMS(2); (c)Ti-HMS(10); (d) P25.
From Figure 3, we can
deduce the yields of the photo-formed N2 and N2O (efficiency)
and their selectivity in the photocatalytic decomposition of NO on various types of
titanium oxide catalysts. It is clear that the efficiency and selectivity for the
formation of N2 strongly depend on the type of catalyst. The Ti-HMS (1)
and Ti-HMS (2) exhibit a high reactivity and a high selectivity for the formation of N2
while the formation of N2O is found to be the major reaction on the bulk TiO2
catalyst. Therefore, the results obtained with Ti-HMS catalysts show some
difference in selectivity as well as efficiency with the difference of Ti-content and the
bulk TiO2 catalyst.
The photocatalytic performance of titanium oxide appears to be
completely modified by its incorporation into HMS, which is associated with changes in the
molecular structure and coordination environments. The
unique catalytic performance of the highly dispersed titanium photocatalyst Ti-HMS(1) in
comparison with higher loading photocatalysts, such as Ti-HMS(10) , shows higher
photocatalytic activity and selectivity for the formation of N2. The pure TiO2
phase is not active for the formation of N2 in the directly decomposition of
NO, while the highly dispersed TiO2 within HMS exhibit high reactivity and high
selectivity for the formation of N2. In the present study, high Ti content
photocatalysts also display low reactivity and selectivity in the decomposition of NO. In
contrast, the molecularly dispersed Ti-HMS(l) photocatalyst is much more active for
decomposition of NO and is highly selective for the formation of N2. In
addition, the catalytic activity and selectivity for the formation of N2
on the Ti-HMS photocatalysts are also a strong function of TiO2
content, which is associated with the changes in the coordination geometry and the degree
of polymerization of the surface Ti atoms. From the results, it can be found
that the photocatalytic activity and selectivity for formation of N2 decrease
with increasing Ti content in the photocatalysts Ti-HMS.
4 CONCLUSIONS
N2 adsorption/desorption measurements have shown that the mesoporous
structures of Ti-HMS become less uniform upon the introduction of Ti into the
framework and the hexagonal lattice will be destroyed. The results also indicate that pore
volume, pore diameter and surface area decrease are mainly caused by increasing Ti-content
in Ti-HMS.
The results show that a high photocatalytic efficiency and selectivity
for the formation of N2 in the photocatalytic decomposition of NO was achieved
with the Ti-HMS(l) catalyst having highly dispersed isolated tetrahedral titanium oxide
species, and decreased with increasing Ti content. The formation of N2O
in the photocatalytic decomposition of NO was found to proceed on the bulk TiO2
catalysts and on the Ti-HMS(10) due to aggregated octahedrally coordinated titanium
oxide species.
¡¡
REFERENCES
[1] Anpo M, Yamashita H., in "Surface Photochemistry", Anpo M., Ed., John Wiley
& Sons: Chichester, U. K., 1996, 117.
[2] Anpo M. Catal. Surv. Jpn. 1997, 1: 169.
[3] Hoffmann M R, Martin S T, Choi W et al. Chem. Rev., 1995, 95: 69.
[4] Fox M A, Dulay M T. Chem. Rev., 1993, 93: 341.
[5] Ollis D F, A1-Ekabi H Eds. Photocatalytic Purification and Treatment of Water and Air.
Elsevieer: Amsterdam, 1993.
[6] Ichihashi Y, Yamashita H, Anpo M. Study. Surf. Sci. Catal., 1997, 105: 1609.
[7] Anpo M, Ichihashi Y, Takeuchi M et al. Res. Chem. Intermed., 1998, 24 (2): 143.
[8] Serpone N, Pelizzetti E. Photocatalysis, Wiley, New York, 1989.
[9] Choi W Y, Hoffmann M R. Environ. Sci. Technol., 1997, 31: 89.
[10] Zhang Z, Wang C C, Zakaria R et al. J. Phys. Chem. B, 1998, 102: 10871.
[11] Yamashita H, Ichihashi Y, Anpo M et al. J. Phys. Chem., 1996, 100: 16042.
[12] Anpo M, Yamashita H. in "Heterogeneous Photocatalysis", Schiavello M Ed.,
Wiley & Sons: London, 1997, 131.
[13] Beck J S, Vartuli J C, Roth W J et al. J. Am. Chem. Soc., 1992, 14: 10834.
[14] Sayari A. Chem. Mater., 1996, 8: 1840.
[15] Corma A. Chem. Rev., 1997, 97: 2373.
[16] Kruk M, Jaroniec M, Sayari A. Langmuir, 1999, 15: 5683.
[17] Ramaan N K, Anderson M T, Brinker C. J. Chem. Mater., 1996, 8: 1682.
[18] Moiler K, Bein T. Chem. Mater., 1998, 10: 2950.
[19] Ying J Y, Mehnert C P, Wong M S. Angew. Chem., Int. Ed. Engl., 1999, 38: 56.
[20] Blasco T, Camblor M A, Corma A et al. J. Am. Chem. Soc., 1993, 15: 11806.
[21] Zhang W, Froba M, Wang J et al. J. Am. Chem. Soc., 1996, 118: 9164.
[22] Sing K S W, Everett D H, Haul R A et al. Pure Appl. Chem., 1985, 57: 60,
[23] Zhang J L, Minagawa M, Agusawa T et al. J. Phys. Chem. B., 2000, 104: 11501.
¡¡
|