Study on the standard
enthalpy of formation of 2-amino-pyrimidine derivative and their manganese complexes
Jiao Baojuan, Wei Qing, Chen
Sanping, Zhang Sumin, Gao Shengli
(Shaanxi Key Laboratory of Physico-Inorganic Chemistry, Department of Chemistry,
Northwest University, Xi'an Shaanxi 710069, China)
Received Jul. 6, 2003; Supported by the
Education Committee of Shaanxi Province for financial support (No. HF01304)
Abstract The complexes of
hydrous manganese chloride with 2-amino-4, 6-dimethyl-pyrimidine (ADMP) and 2-amino-4,
6-dimethoxygenpyrimidine (AMP) were prepared through reflux in alcohol. The compositions
of the complexes Mn(ADMP)2Cl2 and Mn(AMP)2Cl2
were determined by chemical and elemental analyses. The complexes were characterized by
IR, XPS, 1H NMR and TG-DTG techniques. The constant-volume combustion energies
of ADMP, AMP, Mn(ADMP)2Cl2 and Mn(AMP)2Cl2,
were determined by a precise rotating bomb calorimeter at 298.15K. They were (每3664.53 ㊣ 1.18) kJ · mol-1,
(每3340.01 ㊣ 1.54) kJ
· mol-1, (每7043.08 ㊣ 3.13) kJ · mol-1, and (每6058.71 ㊣ 3.18) kJ · mol-1. Their
standard enthalpies of combustion,  , and standard enthalpies of formation,  ,
were calculated. They were (每3666.39 ㊣ 1.18) kJ · mol-1, (每3339.39 ㊣ 1.54) kJ · mol-1, (每7043.08 ㊣ 3.13) kJ · mol-1,
(每6053.75 ㊣3.18) kJ
· mol-1 and (19.09 ㊣ 1.43) kJ · mol-1, (每307.91 ㊣ 1.74) kJ · mol-1,
(每669.90 ㊣ 3.51) kJ
· mol-1, (每1659.23 ㊣ 3.56) kJ · mol-1.
Keywords manganese chloride, 2-amino-4,
6-dimethyl-pyrimidine, 2-amino-4, 6-dimethoxygen-pyrimidine, standard enthalpy of
combustion, standard enthalpy of formation
The pyrimidines as a class are known to
possess extraordinary biological properties that are generally distinguished qualitatively
by their applications in pesticide, herbicide, bactericide, and medicine intermediates [1].
A survey of these applications and a number of the related variations that are recently
developed, such as the extraordinary effective herbicide of sulfonyl sulfourea, reveals
the broad biological importance just because of the wide occurrence of pyrimidine ring
systems in these molecules [2]. It has been shown that the medicine
intermediates in the complexes of metal ions and pyrimidines could prolong the
pharmaceutical activity and effective life, and reduce the damage to mammal [1, 3 -8]. When the complexes of Pt or Pd with pyrimidine are
employed as medicine additives, they are harmful to human body, especially to some organs
such as kidneys, although they are identified as the most effective drugs used to treat
cancer by now. If the roles of Pt and Pd in these complexes were taken by such
microelements that are necessary for life as Cu, Zn or Co, et al, the toxicity and the
side effect of these complexes would be decreased while the power and efficiency of the
medicine are preserved.
In the present work, the
solid complexes of the MnCl2 with 2-amino-4, 6-dimethyl-pyrimidine (ADMP) and
2-amino-4, 6-dimethoxygenpyrimidine (AMP) were prepared. The compositions of these complexes were
determined by chemical and elemental analyses, and the complexes were characterized by IR,
XPS, 1H NMR and TG-DTG techniques. The constant volume-combustion energies of
ADMP (a), AMP (c), Mn(ADMP)2Cl2 (b) and Mn(AMP)2Cl2
(d) were determined by a precise rotating bomb, the standard combustion enthalpies and the
standard formation enthalpies were calculated. Clearly the study of the complexes is of
substantial practical, as well as theoretical significance.
1 EXPERIMENTAL
1.1 Reagents and experimental conditions
All chemicals and solvents were of anal. grade
and used after further purification. Guanidine nitrate, diethyl malonate and acetylpropyl
ketone were made in the Second Branch of The Head Reagent Factory of Shanghai; alcohol,
MnCl2 · 4H2O, phosphorus oxychloride, triethylbenzylamine
chloride, sodium methylate and K2CO3 were purchased from the
Chemical Reagent Factory of Xi'an. ADMP and AMP were synthesized according to Ref. [7, 8]. The yield of white
solid product (ADMP) is 99 %, with the melting point of 152-152.4ºC,better than
152.9-154.4 ºC in the literature [7].Referred to Ref. [9], the synthesis
method of 2-amino-4, 6-dichloro-pyrimidine was ameliorated. The white crystal of AMP was
prepared. The yield of AMP was beyond 80 %, and its melting point locates in the range of
97.0-97.5 ºC.
Melting point of the compounds was measured with WRS-1A digital
melting-point apparatus. Mn2+ content was complexometrically determined by
EDTA, Cl- by the Fajans' method and C, H, N contents were determined on a Perkin 每 Elmer 2400 type elemental
analyzer. The IR spectra (KBr pellets) were obtained on a Bruker EQ UINOX-550
spectrophotometer in the 400 每 4000 cm-1 region. XPS were taken on an ESCA PHI-5400 X-ray
photoelectron spectrophotometer using Mg radiation
X-ray source, the C1s electron in benzene was used as the internal
standard, BE = 284.6 eV, and the accuracy of the measured BE value
was + 0.1 eV. The NMR spectra of the compounds were measured with a Varian
Unity INOVA-400 nuclear magnetic resonance spectrometer using TMS as the reference sample
and C2D5OD as the solvent. TG and DTG data were determined by a
Perkin Elmer thermogravimetric analyzer. All TG-DTG tests were performed under a dynamic
atmosphere of dry nitrogen at a flow rate of 60 mL min-1, the heating rate was
10 deg min-1 and sample masses approximated to 1 mg.
The constant-volume combustion energies of the compounds were
determined by a precise rotating bomb calorimeter [10]. The main experimental
procedures were described in Ref. [11]. The initial temperature was regulated to (25.0000
㊣ 0.0005) ºC, and the initial oxygen pressure was 2.5 MPa. The correct value of the
heat exchange was calculated according to the Linio -Pyfengdelel-Wsawa formula [12]. The calorimeter
was calibrated with benzoic acid of 99.999 % purity. It had an isothermal heat of
combustion at 298.15 K of (-26434 ㊣ 3 ) . The energy equivalent
of calorimeter was determined to be (17936.01 ㊣ 9.08) kJ · K-1. The
analytical methods for final products (gas, liquid, and solid) were the same as those in
Ref. [10].
1.2 Preparation of the complexes
For preparation of the complex b, 6.8577g (0.0557mol) of the ligand and 5.5099g
(0.0278mol) of MnCl2 · 4H2O were weighed out, and separately
dissolved in 50 mL and 25 mL of alcohol. When the solution of MnCl2 · 4H2O
was warmed for a few minutes on a hot plate, the solution of the ligand was added dropwise
into the solution of salt. Under the condition of reflux, the reaction proceeded further
for 4 h. After evaporating a part of solvent, the precipitant appeared, and the reaction
mixture was allowed to cool slowly to room temperature, followed by suction filtration. In
order to remove the excessive ligand and salt from product, it was necessary for the
reaction mixture to be rinsed thoroughly with hot alcohol and distilled water. An infrared
heat lamp was employed to serve the drying of the product. Finally, the pale red product
of the yield of 60 % was obtained. The complex d was prepared according to the
procedures used for the complex b above, showing pale red color and the yield of 72 %. The
purity of the complexes was greater than 99.9% checked by HPLC. The analytical results of
the composition of these complexes are presented as follows. For b, wi
(calc.): 14.76 % Mn, 19.09 % Cl, 38.60 % C, 4.81 % H, 22.58 % N; wi
(found): 14.70 % Mn, 19.15 % Cl, 38.71 % C, 4.80 % H, 22.60 % N. For d, wi
(calc.): 12.60 % Mn, 16.28 % Cl, 33.02 % C, 4.13 % H, 19.27 % N; wi
(found): 12.55 % Mn, 16.33 % Cl, 33.05 % C, 4.18 % H, 19.37 % N. The compositions of the
complexes b and d were identified as Mn(ADMP)2Cl2 and Mn(AMP)2Cl2.
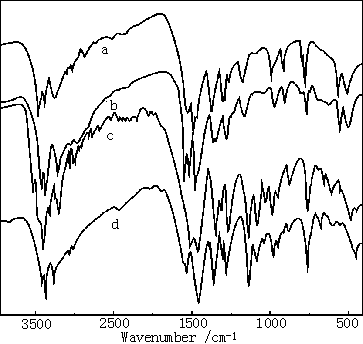
(a)ADMP (b) Mn(ADMP)2Cl2
(c) AMP (d) Mn(AMP)2Cl2
Fig.1 IR Spectra of the ligand and the complexes
2 RESULTS AND DISCUSSION
2.1 IR spectra of the complexes
IR spectra of the ligand and the complexes were
shown in Fig.1. It is obvious from the infrared spectra of the compounds that there are
differences between the main characteristic absorption peaks of the complexes and those of
the ligand [13,14]. Compared with the characteristic absorption peaks of the
ligand, the characteristic absorptions of CˊN and C-N in the ring of pyrimidine, as well as those of
stretching vibration and bending vibration of N-H connected with the ring, have the shifts of 15 cm-1,
18 cm-1, 35 cm-1, and 40 cm-1 for the complex b and those
of 20cm-1, 25 cm-1, 30 cm-1, and 40 cm-1 for
the complex d. Based on the analyses above, it is shown that for the ligand the nitrogen
atom of the amino group and one of the nitrogen atoms of the pyrimidine coordinate to Mn2+
in a bidentate fashion. The characteristic vibrations of Cl- in the complexes
occur in the fingerprint region of 200 每 400 cm-1, which is difficult to show in the
recorder.
2.2 XPS spectra of the complexes
Consulting Ref. [15], the binding energy data of the internal shell electron for the
main atoms of the compounds obtained from XPS spectra of the complexes, are listed in
Table 1.
Table 1 Binding Energy
Data / eV
Compound |
N1s(amino) |
N1s(the ring of pyrimidine) |

|

|
MnCl2·4H2O |
ㄜ |
ㄜ |
199.4 |
642.2 |
a |
399.8 |
398.5 |
ㄜ |
ㄜ |
b |
399.2 |
399.9 |
198.2 |
642.6 |
c |
400.1 |
398.2 |
|
|
d |
399.1 |
399.3 |
198.0 |
643.1 |
Four conclusions could be
drawn from the analyses of the binding data. 1. Compared with the binding energy of N1s
of the amino group and that of N1s of the pyrimidine ring, there are
changes for those of the complexes, which shows that nitrogen atom of the amino group and
one of the nitrogen atoms in the pyrimidine coordinate to Mn2+. The decrease of
the binding energy could be interpreted that rather amount of feedback of d electrons
existing in the coordination bond of N↙Mn2+
leads to the increase of the electron cloud density of N1s. 2. The
binding energies of Cl- have a distinctly changing, showing that Cl-coordinates to Mn2+. 3. For the
complexes b and d, the binding energy of Mn2+ has changes of 0.4 eV and 0.9 eV
and the ranges of the differences are not wide, which illustrates a certain degree of
feedback bond existing in the coordination bond. 4. Combining the analyses of XPS spectra
with the results of IR analyses, it indicates that AMP has stronger coordination to Mn2+
than ADMP, which is credited to the strong donor strength of CH3O-.
2. 3 1H NMR spectra of the compounds
The chemical shifts for the main group of 1H NMR spectra of the ligand and the
complexes are listed in Table 2, showing that two ligands coordinate to Mn2+
for the complexes.
Table 2 Chemical Shifts of the Main
Groups for the compounds
Compound |
Chemical shift d |
a |
2.304 (s, 6H, -CH3) |
6.401(s, 1H, =CH-) |
4.969 (s, 2H, -NH2) |
b |
2.303 (s, 12H, -CH3) |
6.399 (s, 2H, =CH-) |
4.923 (s, 4H, -NH2) |
c |
3.757 (s, 6H, -OCH3) |
5.347(s, 1H, =CH-) |
6.563(s, 2H, -NH2) |
d |
3.760 (s, 12H, -OCH3) |
4.871 (s, 2H, =CH-) |
5.486 (s, 4H, -NH2) |
2. 4 Thermostability of the complexes
Thermostability of the solid complexes was investigated by TG-DTG and the TG curves of
the complexes are depicted in Fig 2. The curves of TG-DTG concerning the compounds reflect
that the experimental results for the residual amount of loss of weight are in good
agreement with the calculated results, and the intermediate and final products of the
thermal decomposition of the complexes are identified by IR spectra as well. The thermal
decomposition processes of the complexes are summarized in Table 3.
Obviously, the complexes b and d
were completely decomposed into manganese chloride after processing skeleton splitting of
part of the ligand.
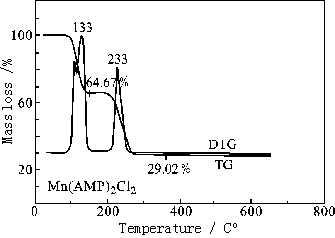 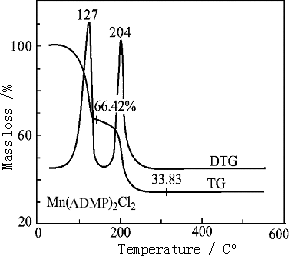
Fig. 2 TG-DTG curves of the complexes
Table 3 Thermoanalytical results of the
complexes
Complex |
Decomposition product |
Decomposition temperature / ºC |
Residual rate / % |
b |
Mn(ADMP)2Cl2 ·MnCl2
MnCl2 |
30-127-146*
146-204-320 |
66.42 (66.91)**
33.83 (33.82) |
d |
Mn(AMP)2Cl2·MnCl2
MnCl2 |
30-133-154
154-233-362 |
64.67(64.43)
29.02(28.85) |
* The
intermediate data were peak temperatures of DTG curves. ** The data in brackets
were calculated values
Table 4 The Experimental
Results for Combustion Energies for a, b,c and d.
Compound |
No |
Mass of sample m/g |
Calibrated heat of combustion wire qc / J |
Calibrated heat of acid containing nitrogen qn /
J |
Calibrated
DT/K |
Combustion energy of sample
 |
a |
1 |
0.80525 |
12.60 |
70.91 |
1.3344 |
29725.72 |
2 |
0.69770 |
12.60 |
61.44 |
1.1567 |
29736.82 |
3 |
0.74586 |
12.60 |
65.68 |
1.2378 |
29768.32 |
4 |
0.78249 |
9.00 |
68.91 |
1.2986 |
29773.94 |
5 |
0.80021 |
12.60 |
70.47 |
1.3266 |
29738.03 |
6 |
0.79233 |
12.60 |
69.77 |
1.3155 |
29782.50 |
mean |
﹛ |
﹛ |
﹛ |
﹛ |
29754.22㊣9.60 |
b |
1 |
1.00310 |
12.60 |
183.80 |
1.0641 |
18899.56 |
2 |
1.01478 |
12.60 |
185.95 |
1.0793 |
18949.54 |
3 |
1.00635 |
12.60 |
184.40 |
1.0695 |
18934.52 |
4 |
1.06526 |
12.60 |
195.20 |
1.1310 |
18916.51 |
5 |
1.04723 |
11.70 |
191.90 |
1.1112 |
18905.86 |
6 |
1.06545 |
12.60 |
195.23 |
1.1328 |
18943.52 |
mean |
|
﹛ |
﹛ |
﹛ |
18924.92㊣8.41 |
c |
1 |
0.77961 |
12.60 |
67.17 |
0.9369 |
21530.39 |
2 |
1.03304 |
12.60 |
89.38 |
1.2400 |
21509.09 |
3 |
1.90079 |
12.60 |
78.28 |
1.0912 |
21560.98 |
4 |
1.00319 |
12.60 |
86.60 |
1.2038 |
21501.25 |
5 |
1.01272 |
12.60 |
87.42 |
1.2179 |
21509.37 |
6 |
1.00303 |
12.60 |
86.58 |
1.2040 |
21509.15 |
mean |
|
|
|
|
21526.70㊣9.95 |
d
﹛
﹛
﹛ |
1 |
1.15142 |
12.60 |
187.67 |
0.9002 |
13899.23 |
2 |
1.04970 |
12.60 |
171.15 |
0.8201 |
13888.38 |
3 |
1.06786 |
12.60 |
174.12 |
0.8362 |
13920.81 |
4 |
1.10235 |
12.60 |
179.75 |
0.8601 |
13870.42 |
5 |
1.11249 |
12.60 |
181.40 |
0.8693 |
13891.38 |
6 |
1.11533 |
12.60 |
181.86 |
0.8706 |
13876.57 |
mean |
|
|
|
|
13891.15㊣7.30 |
2. 5 Combustion Energy of the compounds
Standard enthalpies of formation of ligand and complexes are studied. The determination
method of constant volume combustion energy for the sample is the same as the calibration
of the calorimeter with benzoic acid. The combustion energies of the samples are
calculated by the formula
(1)
where (sample, s) denotes the constant
volume combustion energy of the samples, W is the energy equivalent of the RBC-III
type calorimeter (in J · K-1), DT the correct value of the temperature
rising, a the length of actual Ni -Cr wire
consumed (in cm), G the combustion enthalpy of Ni - Cr wire for ignition (0.9 J · cm-1), 5.983 the
formation enthalpy and solution enthalpy of nitric acid corresponding to 1 cm3
of 0.1000 mol· cm-3 solution NaOH (in J · cm-3), b
the volume in cm3 of consumed 0.1000 mol· dm-3 solution of
NaOH and m the mass in g of the sample. The results of the calculations are
given in Table 4.
2. 6 Standard Combustion Enthalpies of the
Compounds
The standard combustion enthalpy of the compounds, , is referred to the combustion enthalpy change of the following ideal
combustion reaction at 298.15 K and 101.325 kPa.
C6H9N3 (s) + O2 (g) = 6CO2 (g) + H2O (l) + N2
(g) (2)
C6H9N3O2 (s) + O2 (g) = 6CO2 (g) + H2O (l) + N2
(g) (3)
Mn(ADMP)2Cl2 (s) + 17 O2 (g) = MnO2 (s) + 12
CO2 (g) + 8 H2O (l) + 2 HCl (g) + 3 N2 (g) (4)
Mn(AMP)2Cl2 (s) + 15O2 (g) = MnO2 (s) + 12 CO2
(g) + 8 H2O (l) + 2 HCl (g) + 3 N2 (g) (5)
The standard combustion enthalpies of the
samples are calculated by the following equations
(6)
(7)
where ng is the total
amount of substance (in mole) of gases present as products or reactants, k = 8.314
J · mol-1 ·K-1, T = 298.15 K. The
results of the calculations are given in Table 5.
Table 5 Combustion
Energies, Standard Enthalpies of Combustion and Standard Enthalpies of Formation for a,
b, c and d
Compound |
/(kJ·mol-1) |
 /(kJ·mol-1) |
 /(kJ·mol-1) |
a |
3664.53㊣1.18 |
3666.39㊣1.18 |
-19.09㊣1.43 |
b |
7043.08㊣3.13 |
7043.08㊣3.13 |
669.90㊣3.51 |
c |
3340.01㊣1.54 |
3339.39㊣1.54 |
307.91㊣1.74 |
d |
6058.71㊣3.18 |
6053.75㊣3.18 |
1659.23㊣3.56 |
2.7 Standard Enthalpies of Formation of the
compounds
The standard enthalpies of formation of
the samples,  , are calculated by Hess's law according to
the following thermochemical equations
(8)
(9)
(10)
(11)
where  = 每 530.03 kJ · mol-1,  = (每 393.51㊣0.13) kJ ·
mol-1,
 = (每 285.83 ㊣
0.042) kJ · mol-1,  = (每 92.31 ㊣ 0.03) kJ · mol-1 [16].
The detailed list of the results of the calculations is presented in
Table 5.
As described in Table 5, thermo stabilizations of the complexes
decrease in the order Mn(AMP)2Cl2, Mn(ADMP)2Cl2,
AMP and ADMP, which is related to the structures of the substances.
REFERENCES
[1] Wu J, Gong Y Q. Chin. J. Appl. Chem. (Yingyong Huaxue), 2000, 17 (5): 558.
[2] Liu C L. Pesticides. (Nong Yao), 1995, 34 (8): 25.
[3] Zhang P Z, Gong Y Q, Shi X Q. Chem. Res. and Appl. (Huaxue Yanjiu & Yingyong),
1997, 9 (1): 92.
[4] Mishra L K. J. Indian Chem. Soc. 1982, 59 (3): 408.
[5] Rochon F D. Can. J. Chem. 1979, 57 (5): 526.
[6] Allan J R. J. Therm. Anal. 1981, 22 (1): 3.
[7] Kong F L. Chemical world, (Huaxue Shijie), 1991, 32 (6): 254.
[8] Xu K X, Handbook of Fine Industrial Chemicals and Intermediate. Beijing: Beijing
Chemical and Industrial Press, 1999, (4): 100-101.
[9] Li L, Wei Q, Gao S L, Zhang P, Shi Q Z. J. Northwest Univ. (Natural Sci. Edi.). 2001,
19 (9): 917.
[10] Liu J R, Yang X W, Hou Y D, et al. Thermochim. Acta. 1999, 329: 123.
[11] Yang X F, Yang X W, Sun L Z. Chem. J. Chin. Univ. (Gaodeng Xuexiao Huaxue Xuebao),
1986, 7: 363.
[12] Popov M M. Thermometry and Calorimetry. Moscow: Moscow University, Publishing house,
1954, 382.
[13] Dong Q N. Infrared Spectrometry. Beijing: Beijing Chemical and Industrial Press,
1979.
[14] Nakamoto K. (Huang D R, Wang R Q. Translated) Infrared and Raman Spectra of Inorganic
and Coordination Compounds. Beijing: Beijing Chemical and Industrial Press (4th Edi),
1991.
[15] Wang J Q, Wu W H, Feng D M. Instruction to X-Ray Photoelectron spectroscopy
(XPS/XAES/UPS). Beijing: Beijing National Defence Industry Press, 1992.
[16] Cox J D. J. Chem. Thermodyn. 1978, 10: 903.
﹛
|