Melting behavior and
nonisothermal crystallization kinetics of metallocene polyethylene
Gao Jungang, Li Shurun, Li Zhiting
(Department of Polymer Science, College of Chemistry and Environmental Science, Hebei
University, Baoding 071002, China)
Received July 29, 2003; Supported by the
National Natural Science Foundation of China (No.201068) and Educational Science
Foundation of Hebei (No.2000105)
Abstract Melting behavior and
nonisothermal crystallization kinetics of Metallocene Polyethylene (mPE-D65) was studied
using differential scanning calorimetry (DSC) at various scanning rates. The Avrami
equation modified by Jeziorny and a method developed by Mo were employed to describe the
nonisothermal crystallization process of mPE. The theory of Ozawa was also used to analyze
the mPE DSC data. Kinetic parameters such as the Avrami exponent (n), the
crystallization rate (Zc), the peak temperatures (Tp)
and the half-time of crystallization (t1/2) etc. could obtain at various
scanning rates. The results showed that the Ozawa analysis failed to provide an adequate
description of the nonisothermal crystallization of mPE. The Avrami equation modified by
Jeziorny and a method developed by Mo were fit for describing the nonisothermal
crystallization process of mPE. The value of n showed that the nonsiothermal
crystallization of mPE corresponds to a tridimensional growth with heterogenous
nucleation, and the value of Zc increase with increasing of the cooling
rates for mPE.
Keywords Metallocene Polyethylene; Melting behavior; Nonisothermal crystallization
kinetics
1 INTRODUCTION
Polyethylene (PE) [1] is the most widely used commercial polymer in the world
today. Because of its specific properties, such as high chemical and mechanical
resisitance, low specific gravity and cost, the industrial PE market is still growing.
Metallocene Polyethylene (mPE) is a linear polyethylene which catalyzed by metallocene.
mPE offers the following significant performance advantages compared with conventional
polyethylene: (1) tensile, puncture and impact
performance improvements, providing superior toughness; (2) sealing performance
improvements; (3) easy blending with other
polyolefins.
Investigations of the kinetics of polymer crystallization are
significant both theoretically and practically. Most frequently, the investigations are
conducted under isothermal conditions because of the convenience of the theoretical
treatment of the data. Practically mPE usually undergoes a nonisothermal crystallization
environment in the processing. So a study on the nonisothermal crystallization process of
mPE is meaningful, but it is so less about this investigation until now.
In this article, the nonisothermal crystallization process of mPE were
investigated by modified Avrami equation and a method developed by Mo Z.S. The theory of
Ozawa was also used to analyze the mPE DSC data at different scanning rates [2].
2 EXPERIMENTAL
2.1 Materials
The mPE was supplied from Exxon Chemical Company (USA) as an exact grade D65.
(MI=0.10g/min; denstiy=0.918g/cm3; melting point=119ºC)
2.2 Thermal Measurements
The weight of all samples was kept in approximately 10mg in DSC measurement by using a
CDR-4P calorimeter under a nitrogen atmosphere. The temperature and melting enthalpy were
calibrated with standard indium. In order to erase the previous thermal history, the
samples were encapsulated into aluminum pans and were heated to 180ºC and kept at this
temperature for 5 minutes. They were then cooled to room temperature at different constant
cooling rates (D) (namely D=5,8,10,12,15ºC/min, respectively) and
the heat flow during crystallization was recorded as a function of time or temperature.
For heating process, the samples that had been erased thermal history were heated at
different constant rates (namely D=5,8,10,12,15ºC/min, respectively) from
room temperature to 180ºC again, and the heat flow was recorded.
3 RESULTS AND DISCUSSION
3.1 Theories of Kinetic Parameter Determinations
The relative crystallization (Xt), [3] as a function of temperature
is defined as:
(1)
where and
are the onset and end of crystallization temperature, respectively. is the heat flow at temperature T.
The time of the fastest crystallization (tmax) is the
time of crystallization starts to the appearance of crystallization peak. As a function of
temperature (T) and cooling rate (D), tmax is defined as:
(2)
where Tp is the temperature of crystallization peak.
The half-time of crystallization (t1/2) [4]
is the time required for 50% crystallization.
Sample crystallinity (Xc) [5] is defined
as:
(3)
where and
are the melting enthalpy of PE sample and 100% crystallization PE, respectively, where =273J/g [6]. is acquired by the integral area under the DSC heating curve.
The kinetic parameters of nonisothermal crystallization were
determined, based on the simplified assumption that crystallization occurs under constant
temperature. In this case, the Avrami equation can be used [7-8]:
(4)
or (5)
where Xt is the relative crystallinity at time t, t can be
obtained by the equation (6).
(6)
where T is the temperature at crystallization time t, and D is the
cooling rate. From Eqs.(5) and (6), we have equation (7):
(7)
where n is the Avrami exponent, which depends on the type of nucleation and growth
dimension, and the parameter Zt is a composite rate constant that
involves both nucleation and growth rate parameters. The Avrami exponent (n) and
crystallization rate (Zt) can be obtained from the slope and intercept
of the line in the plot of versus . Considering the effect of the cooling rate, Zt
is corrected by cooling rate [9]:
(8)
where Zc is the kinetic crystallization rate.
Ozawa [10] had extended the Avrami equation to the
nonisothermal condition. Assuming that the nonisothermal crystallization process may be
composed of infinitesimally small isothermal crystallization steps, the following equation
was derived:
(9)
or (10)
where K (T) is the function of cooling rate, m is the Ozawa exponent,
which is depended on the dimension of the crystal growth.
A method developed by Mo [11] was employed to describe the
nonisothermal crystallization for comparison. For the process, physical variables relating
to the process are the relative crystallization Xt, cooling rate D,
and crystallization temperature T. Both the Ozawa and Avrami equation can relate to
these variables as follows:
(11)
and by rearrangement:
(12)
where refers to the cooling rate value, which must
be chosen within unit crystallization time when the measured system amounts to a certain
relative crystallization; a is the ratio of the Avrami exponent n to the
Ozawa exponent m (a=n/m). According to eq. (12), at a given
relative crystallization, by plotting log D versus log t it yields a
linear relationship between log D and log t. The data of kinetic
parameter F (T) and a can be estimated from the intercept and slope.
3.2 Melting and Crystallization Behavior of mPE
Figure 1 shows DSC curves of mPE at different heating rates. From these curves, some
useful data for describing their nonisothermal melting behavior can be obtained, such as
the melting curve shape and peak temperature (Tp) at which mPE has the
fastest melting rate, melting enthalpy ( ) and
crystallinity (Xc). The values of the parameters determined are given in
Table 1. From these melting curves we can see that there are two melting peaks during
different heating rates, it showed that this mPE (D65) molecules used has not homogenizing
tacticity and grafting chain distribution in the molecules, some molecules on chains
segment have not good tacticity that will be melting earlier and have a higher degree of
graft, and the other later, which have a good tacticity and a less degree of graft.
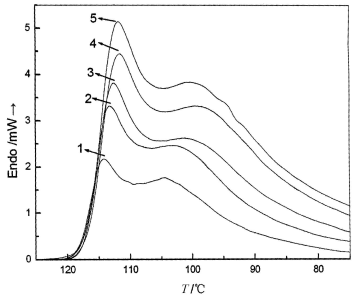 |
Fig.1 DSC curves of mPE at
different heating rates.
1-5ºC/min; 2-8ºC/min; 3-10ºC/min; 4-12ºC/min; 5-15ºC/min |
This fact can be also
showed from the crystallization exotherms process of this mPE at different cooling rates
which are showed in Figure 2. As seen from the figure 2, we can also see that there are
two peaks during the nonisothermal crystallization of mPE.From these curves, some useful
data can be obtained for describing their nonisothermal crystallization behavior, such as
the peak temperature (Tp), relative crystallinity (Xt),
the half-time of crystallization (t1/2), and the crystallization rate (Zc)
etc. And the values of the parameters determined are given in Table 2. From Figure 2, we
can see that Tp moves to a lower time scale that allows the polymer to
crystallize as the cooling rate increasing, therefore requiring a higher power to initiate
crystallization. It is clearly seen from Table 2 and Figure 2; the changement of
parameters for this mPE at different cooling rates is corresponding to that at different
heating rates. Most of molecules which has a higher tacticity and less degree of graft
begin to crystallize at higher temperature whereas the others at lower temperature.
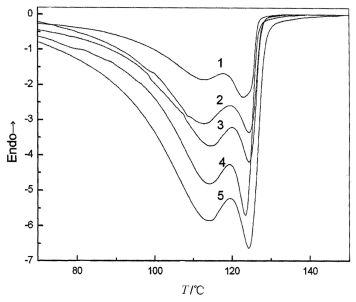 |
Fig. 2 DSC curves of mPE at
different cooling rates.
1-5ºC/min; 2-8ºC/min; 3-10ºC/min; 4-12ºC/min; 5-15ºC/min |
Table 1 Parameters
of mPE Sample during Nonisothermal heating Process
D ( ºC/min) |
5 |
8 |
10 |
12 |
15 |
Tp1 ( ºC) |
112.5 |
112.6 |
114.5 |
114.5 |
114.5 |
Tp2 ( ºC) |
123.0 |
124.6 |
124.5 |
123.6 |
124.5 |
¦¤Hf (J/g) |
136.7 |
140.9 |
137.5 |
130.4 |
149.0 |
Crystallinity (%) |
50.1 |
51.6 |
50.4 |
47.8 |
54.6 |
Table 2 Parameters of mPE
Sample during Nonisothermal Crystallization Process
D ( ºC/min) |
5 |
8 |
10 |
12 |
15 |
Tp1 ( ºC) |
114.0 |
113.1 |
113.0 |
112.0 |
112.0 |
Tp2 ( ºC) |
104.4 |
103.5 |
102.5 |
99.5 |
100.0 |
tmax (S) |
132.0 |
89.3 |
75.0 |
65.0 |
52.0 |
t1/2 (S) |
246.6 |
156.1 |
123.7 |
102.7 |
82.6 |
n |
2.85 |
2.88 |
2.86 |
2.83 |
2.86 |
log (-Zt) |
-1.908 |
-1.356 |
-1.057 |
-0.821 |
-0.555 |
Zc |
0.415 |
0.677 |
0.784 |
0.854 |
0.918 |
3.3 Nonisothermal Crystallization Kinetic of
mPE
Figure 3 shows a good linear relationship when log [-ln(1-Xt)] is
plotted versus log[(T0-T)/D] for mPE at each cooling rate.
Two adjustable parameters, Zt and n, can be obtained by a linear
regression. The Zt and n parameters are not the same physical
meaning as in the isothermal crystallization, because the temperature changes constantly
in nonisothermal crystallization. This affects the rates of both nuclei formation and
spherulite growth ascribed to their temperature dependence. Therefore Zt
must be calibrated by Jeziorny method. The results are listed in Table 2. It is clearly
seen from Table 2, the value of Zc increase with increasing cooling
rate. The Avrami exponent (n), depends on the type of nucleation and growth
dimension. If the crystallization corresponds to a tridimensional growth with homogenous
that n=3+1=4, then nucleation rate increases with time, and if with heterogeneous n=3+0=3,
the nucleation rate have nothing to do with time [12]. From Table 2, the value
of n is not changing almost with the changement of the cooling rates, it suggested
that the nonisothermal crystallization of mPE corresponds to a tridimensional growth with
heterogeneous nucleation. It showed that this mPE has some molecules which have higher
molecular weight, have an action of nucleation.
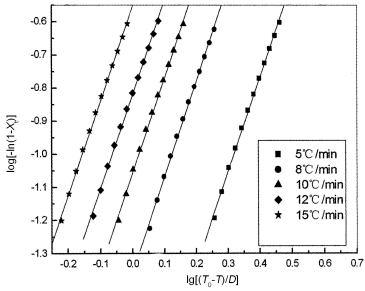
Fig. 3 Plots of log[-ln(1-Xt)] versus log[(T0-T)/D]
for crystallization of mPE |
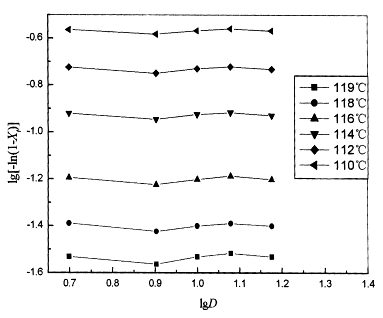
Fig. 4 Ozawa plots of log[-ln(1-Xt)] versus logD
for crystallization of mPE at different crystallization temperatures |
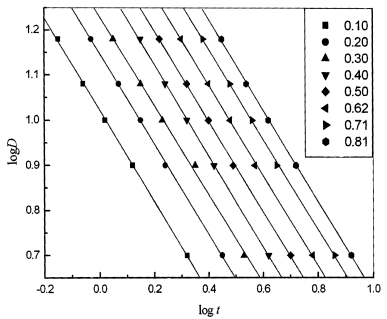 |
Fig. 5 Plots of log
D versus log t for mPE at each given relative degree of crystallization |
Figure 3 shows the results
for mPE according to Ozawa's method. The curvature in Figure 4 presents an accurate
analysis of nonisothermal crystallization data. When crystallization temperature is lower
than 114ºC, this can be explained that, at a given temperature, the
crystallization process at different cooling rates are different stages, that is, the
lower cooling rate processes is toward the end of the crystallization process, whereas at
the higher cooling rate, the crystallization process is an early stage. Although Ozawa's
approach can be used to describe the nonisothermal crystallization behavior of mPE, the
changement in the slope with temperature [Fig.4] means that the parameter m is not
a constant during crystallization, and indicated that Ozawa's approach is not fit for describing the nonisothermal
crystallization process of mPE.
The method developed by Mo.Z.S was also
employed to describe the nonisothermal crystallization for comparison. According to eq.
(12), at a given degree of crystallization, plotting logD versus logt
(Fig.5) yields a linear relationship. The data of kinetic parameter F (T)
and an estimated from the intercept and slope for mPE are listed in Table 3. It can be
seen from Table 3 that F (T) systematically increases with the increase in
the relative degeree of crystallization. It is clear that this approach is successful in
describing the nonisothermal process of mPE.
Table 3 Nonisothermal
Crystallization Parameters of mPE Sample
Xt |
0.10 |
0.20 |
0.30 |
0.40 |
0.50 |
0.62 |
0.71 |
0.81 |
a |
1.015 |
1.005 |
0.987 |
1.015 |
1.005 |
1.005 |
1.005 |
1.015 |
log [F(t)] |
1.023 |
1.149 |
1.231 |
1.327 |
1.400 |
1.480 |
1.561 |
1.632 |
F (t) |
10.54 |
14.09 |
17.02 |
21.23 |
25.12 |
30.20 |
36.39 |
42.85 |
4 CONCLUSIONS
The mPE (D65) molecular chain has not a homogenizing tacticiy, and has two melting
peaks and two crystallization peaks. The Ozawa analysis failed to provide an adequate
description of the nonisothermal crystallization of mPE through of the comparisons of
different stages of crystallization at different cooling rates. The Avrami analysis
modified by Jeziorny and a method developed by Mo.Z.S were successful in describing the
nonistothermal crystallization process of mPE. That the value of n is hardly
changing with the changement of the cooling rates suggested that the nonisothermal
crystallization of mPE corresponds to a tridimensional growth with heterogeneous
nucleation, and the value of Zc increases with increasing cooling rates
for mPE.
REFERENCES
[1] Mori. H, Ohnishi. K, Terano. M. Macromol. Chem. Phys, 1998, 199: 393.
[2] Liu Z H. Introduction to Thermal Analysis. Beijing: Chemical Industry Publishing Co.,
1991: 2.
[3] Kong X H, Yang X N. Euro. Polym. J., 2001, 29(3): 2.
[4] Zhang Z Y, Wu S Z, Du Y H, et al. Chin. J. Polym. Sci., 1991, 9(4): 319.
[5] Dong Y M. The Practical Analyze Technology of Polymer Journal 2001, 37: 1855.
[6] Dong Y M. The Practical Analysis Technology of Polymer Meterials(Gaofenzi Cailiao
Shiyong Poxi Jishu). Beijing: Petrochemical Industry Press, 1979: 293.
[7] Xu R M, Jun T X, Lin S, et al. J. of Appl. Polym. Sci., 2001, 80: 124.
[8] Xu W, Ce M, He P. J. of Appl. Polym. Sci., 2001, 82: 2281.
[9] Jeziorny. A. Polym., 1978, 19: 1142.
[10] Ozawa. T. Polym., 1971, 12: 150.
[11] Liu, T X, Mo Z S, Wang S E, et al. Polym. Eng. Sci., 2001, 82: 2281
[12] He M J, Chen W X, Dong X X, Polymer Physics (Gaofenzi Wuli). Shang Hai: Fudan
Publisher, 1990: 71.
¡¡
|