Thermal properties of
boron-bitrogen containing phenol-formaldehyde resin
Su Xiaohui, Gao Jungang, Jiang Chaojie
(Department of Polymer Science, College of Chemistry and Environmental Science, Hebei
University, Baoding 071002, China)
Abstract The
boron-nitrogen containing phenol formaldehyde resin (BNPFR) was synthesized from phenol,
formaldehyde, boric acid and ammonia water. The thermal properties of BNPFR were
determined by the methods of torsional braid analysis (TBA) and thermogravimetric analysis
(TGA). The results show that BNPFR have a Tg about 210ºC and good thermostability. The thermal degradation kinetics of BNPFR was
investigated by TGA. It is illustrated that the thermal degradation reaction of the BNPFR
follows first-order mechanism, and the half-weight loss temperature is about 150ºC higher
than general phenol-formaldehyde resin.
Keywords Phenol-formaldehyde resin, Boric acid, Nitrogen, thermal analysis
1. ITRODUCTION
Phenolic formaldehyde resins (PFRs) are used principally in the reinforced thermosetting
materials. To improve the flame retardancy and thermo-oxidative resistance of PFR, the
addition of boron has been reported [1-4].The boron- containing
phenol-formaldehyde resin (BPFR) is a modified phenolic resin, which is obtained by
introducing boron to the main chain of common phenol formaldehyde resin. The BPFR
possesses many excellent performances, such as thermostability, mechanical strength,
electric properties and defence of neutron radiation. But the general BPFRs are extremely
sensitive to moisture. To improve the hydrolytic resistance of BPFR, the resins of B-N
coordination bond have been synthesized [5,6], but the description of thermal
property and degradation kinetics has been lacking until now.
The glass transition temperature (Tg) can be used
effectively to illustrate the curing reaction process and thermal properties. In this
work, the BNPFR was synthesized, the torsional braid analysis (TBA) was used to determine
the Tg of the BNPFR, and thermogravimetric analysis (TGA) was used to
study the mechanism and kinetics of thermal degradation.
2. EXPERIMENTAL
2.1 Material
Phenol, boric acid, formaldehyde solution (37% w/w), oxalic acid, 25% ammonia
water, toluene, n-butyl alcohol and para-formaldehyde were all analytically pure
grades and were supplied by the Tianjin Chemical Reagent Co,China.
2.2 Synthesis of BNPFR
BNPFR used in this work was synthesized according to the literature [5,6],Changing
the ratio of boron and ammonia, the resins with different boron- nitrogen ratio were
obtained. Material proportion and the serial number were listed in Table 1.
Table 1 Material Proportion
and Serial Number of BNPFR
Composition |
Phenol : Boric :
Ammonia
(mol ratio) |
Resin. |
Common PR |
17 : 0 : 0 |
1.0N |
17 : 5.5 : 1.0 |
2.2N |
17 : 5.5 : 2.2 |
5.5N |
17 : 5.5 : 5.5 |
The molecular structure of
BNPFR has the following approximate form:
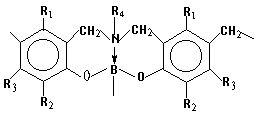
2.3 Torsional braid analysis (TBA)
Specimens, prepared by dipping heat-cleaned glass fiber braid in the tetrahydrofuran
solution of the BNPFR, were completely evaporated in vacuum.
1. The air oven was first heated up to a
desired temperature and kept for a certain period of time until the system reached the
equilibrium state. Specimens were quickly set into the thermostatic baths at a curing temperature
Tc between 140 and 220 ºC. After curing for 1h, respectively, they were taken out
and cooled to room temperature, then their Tgs were determined by TBA
with a heating rate of 2ºC/min.
2. Uncured resin was inserted into the GDP-4 TBA analyzer to determine the available
curing temperature of different boron-nitrogen proportion resins.
2.4 TGA measurements
After curing at 180ºC for 4h, the thermal analysis was carried out on the
Shimadzu DT-40 thermogravimetric analysis (TGA). About 8mg of the sample, which had been
completely cured and ground to fine powers, was put into a platinum cell and placed on the
detector plate, then the furnace was heated at a heating rate of 10ºC/min to 800ºC.
3. RESULTS AND DISCUSSION
3.1 Tg value and the curing process
Generally, the Tg of a
crosslinked resin system is related to the conversion, which depends upon the curing
conditions, such as temperature and time. With these curing condition varying, the Tg
of the system will be changed. So that Tg has been used
directly as a parameter for conversion analysis of curing reaction. Because of the Tg
of thermosetting resin is easy to be measured by TBA, it is particularly useful for the
measurement at high conversion and after vitrification. In this paper, the Tg
values are measured for the BNPFR specimen cured isothermally at different temperatures
for various periods. Different glass transition behaviors occurred for the 2.2N resin
samples with different degrees of curing temperature were shown in Table
2. As seen from Table 2, the BNPFR cured at lower temperature have a low Tg
compared with those cured at higher temperature. It is because that during the curing
process, cross linking reaction has occurred and the weight-average molecular weight
increases, the crossing linking density increases and the mobility of the chains
decreases, the chances for the molecules to collide with each other are reduced and the
intermolecular reaction at this low temperature is limited. But the samples have a higher
curing degree at higher temperature and the Tg value get higher. If the
resin was cured at 220 ºC, there is no loss modulus peak appeared in
TBA curves from 20ºC to 280ºC,
this is due to that BNPFRs have good heat-resistance.
Table 2 The glass transition
temperature of 2.2N resin curing at different temperature for 1h
Curing temperature (ºC) |
140 |
160 |
180 |
200 |
220 |
Tg (ºC) |
150 |
177 |
187 |
211 |
£ |
To determine the influence of boron-nitrogen proportion on
Tgs, the TBA experiment of uncured resins were carried out, the results
are shown in Fig.1, where E is the modulus and F is the mechanical loss tangent. As seen
from Fig.1, at low temperature, the resin has not been cured, the modulus is low, and with
the temperature rising the resin begins to be cured, the modulus increases. When the
temperature approaches a finite value, the resin was completely cured, the modulus does
not change during a certain temperature range. From Fig.1 and Table 3, it can be seen that
there are the same Tg to BNPFRs of different boron-nitrogen proportion,
which illustrate that nitrogen content basically have no influence on Tg
of BNPFRs.
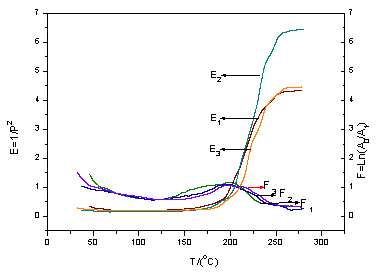
Fig.1 The TBA curve of BNPFR:
(1) 1.0N, (2) 2.2N, (3) 5.5N, at heating rate of 2ºC/min.
Table 3 the Tg
of different resins cured at 200ºC for 1h
Resin |
1.0N |
2.2N |
5.5N |
Tg(ºC) |
214 |
211 |
214 |
3.2 Thermal stability and
degradation kinetics
It can be seen from Fig.2, that common formaldehyde resin (PFR) has higher weight loss
rate than boron-nitrogen containing phenol-formaldehyde resin. The weight loss for common
PFR is over 90% at 600ºC, while the BNPFR is only 32% at the same temperature. The start
temperature of weight loss is at about 420ºC, higher than that of common PFR, and the temperature of
half-weight loss is about 150ºC higher than that of PFR. It is due
to the formation of phenol borate linkage, which reduces the formation of ether linkage,
so that the heat- resistance of BNPFR has increased greatly.
As can be seen from Fig.2, the resins with B-N containing have the same
weight loss before 320ºC, which is about 3%. We deem that it is caused by the evaporation
of water and small molecules and it has not been considered as the degradation kinetics.
The weight loss of BNPFR resins increased obviously from 420ºC, but the
common resin decomposed distinctly from 320ºC, which indicates that the heat-resistance of common resin
is inferior to BNPFR. According to the TGA curves (Fig.2), the degradation process can be
divided into two stages. In the first stage (about 420-600ºC), the weight
loss is about 20%, it may be caused by the oxidation and breakage of some ether linkages
and carbonyl groups. In the second stage (about 600-800ºC), the weight loss is about 55%, it is due to most ether
linkage, carbonyl group, some borate B-O linkage and benzene ring may be oxidized and
broken in this stage.
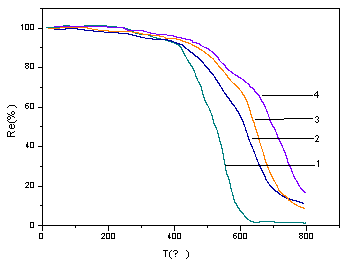
Fig.2 Thermogravimetric
analysis of PFR (1) common PFR, (2) 5.5N, (3) 2.2N, (4) 1.0N, at heating rate of 10ºC/min in air.
To determine the kinetic
parameters of the decomposition from the thermo- gravimetric data, the first step is to
evaluate the conversion of the reaction. In dynamic TGA experiments, the weight change of
the sample is regarded as a function of temperature and the conversion can be expressed as
Eq. (1)
where is the sample
weight in i stage, is the residual
weight of at temperature T.
Therefore, with TGA curves and Eq. (1), the conversions are calculated for different
degradation stages [7,8].
In this work, considering the multi-stage degradation process of BNPFR,
the TGA data were analyzed on the basis of the Madhusdanan-Krishnan-Ninan method [8],
which can be expressed by the following equation:
Eq. (2)
Where A is the pre-exponential factor in the Arrhenius
equation, E is the apparent activation energy, R is the universal gas
constant, f is the heating rate, T is absolute temperature, and is the integral form of the conversion
dependence function. Where . The correct
form of depends on the proper mechanism of
the decomposition reaction [8]. Different expressions of for some solid-state reaction mechanisms can be
described as the following: in first order, is ;
in second order, is ; in third order, is .
According to the above
equations, the activation energy can be obtained at different heating rates from fitting versus plots. For different degradation stages, the apparent activation energies
and pre-exponential factors were all tested for different mechanism functions. Some
results are listed in Table 4.
Table 4 The
kinetic parameters of thermal degradation of 2.2N resin for different mechanism function
at 10ºC/min heating rate
2.2N resin
|
Reaction order |
Correlation coefficient |
Standard deviation |
DE(kJ/mol) |
First stage
|
1
|
0.99435 |
0.14963 |
176.44 |
2
|
0.92303 |
0.34783 |
105.07 |
3
|
0.93041 |
0.6974 |
222.91 |
Second
stage |
1
|
0.99339 |
0.18899 |
255.23 |
2
|
0.96389 |
0.23082 |
208.28 |
3
|
0.96613 |
0.46238 |
421.55 |
As shown in Table 4, for
the same degradation stage at a given heating rate, the correlation values for different
mechanisms are different. According to the principle that the probable mechanism has high
correlation coefficient value and low standard deviation value, the probable mechanism
functions are deduced from the calculated results: the two degradation stages are all
following first order mechanism. The apparent activation energies in each stage are listed
in Table 5.
Table 5 Apparent activation
energy of thermal degradation
DE(kJ/mol) |
PFR |
5.5N |
2.2N |
1.0N |
First stage |
130.04 |
153.48 |
176.44 |
279.93 |
Second stage |
130.01 |
253.31 |
255.23 |
300.87 |
From the data above, we
can conclude that heat-resistance of BNPFR is better than that of common PFR. The
heat-resistance decreased step by step with the increase of nitrogen content, this is due
to the increasing of C-N bond content, which has a lower heat-resistance than B-O bond.
4. CONCLUSION
1. TBA can be used effectively to study the curing process of BNPFR and determine Tg.
2. The N content has little influence on Tg and the proper curing
temperature of BNPFR is 200ºC. The Tg of BNPFR is at about 210ºC.
3. The modulus of the resin increases with the curing temperature increasing, and holding
a certain value during a temperature range.
4. The thermal degradation process of BNPFR can be divided into two stages, and the
thermal decomposition kinetics all follows the first reaction order.
5. The thermo-stability of BNPFR is better than common PR.
REFERENCES
[1] Gao J G, Liu Y F, Wang F L. Eur. Polym. J, 2001, 37: 207-210.
[2] Liu Y F, Gao J G. Polymer Degradation and Stability, 2002, 77: 495-501.
[3] Pitts A. Flame Retardancy of Polymeric Material. New York. Marcel Dekker. 1973.
chapter 2.
[4] Gerrard W. The organic chemistry of boron. London and New York: Academic Press, 1961,
chapter 17.
[5] Heefel H B, Kiessling HJ, Lamper F, Schonrogge B. Ger.Offen 2436358, 1975.
[6] Heefel H B, Kiessling HJ, Lamper F, Schonrogge B. Ger.Offen 2436359, 1975.
[7] Liu Z H. Introduction of thermal analysis. Beijing: Chemical Industry Publishing Co.
1991, 100-110.
[8] Madhusudanan P M, Krishnan K, Ninan K N. Thermochim. Acta., 1986, 97: 189-201.
¡¡
|