Kinetics and mechanism of
oxidation of L-Aspartic acid by dihydroxydiperiodatoargentate (III) in alkaline medium
Shan Jinhuan, Li Shengmin, Huo Shuying ,Shen
Shigang, Sun Hanwen
(Key Laboratory of Analytical Science of Hebei Province, College of Chemistry and
Environmental Science, Heibei University, Baoding 071002, China)
Abstract The kinetics of
oxidation of L-Aspartic acid (ASP) by dihydroxydiperiodatoargentate(III)
(DPA) was studied spectrophotometrically between 25ºC and 45ºC in alkaline medium. The reaction rate showed first order dependence in oxidant
and fractional order in reductant. A plausible mechanism involving a pre-equilibrium of
adduct formation between the complex and reductant was proposed. The rate equations
derived from mechanism can explain all experimental observations. The activation
parameters along with rate constants of the rate-determining step were calculated.
Keywords dihydroxydiperiodatoargentate (III) L-Aspartic acid (ASP) redox reaction,
kinetics and mechanism
Recently, the study of the highest oxidation
state of transition metals has intrigued many researchers. Transition metals in a higher
oxidation state generally can be stabilized by chelation with suitable polydentate
ligands. Metal chelates such as diperiodatoargentate(III)[1],
ditelluratoargentate(III)[2], diperiodatonickelate(IV)[3] are good
oxidants in a medium with an appropriate pH value. The use of complexes as good oxidizing
agents in analytical chemistry has been reported[4].The oxidation of a number
of organic compounds and metals in lower oxidation state by Ag(III) has also been
performed[1], but no further information on the kinetics is available. Because
Ag(III) is in the highest oxidation state and the reaction is complicated in this kind of
reaction system, it is of significance to have a further study on this kind of reaction
system. Investigation on them will certainly provide us with more dynamical parameters,
and will provide theoretical foundation for the design of reaction route in the organic
synthesis and quantitative analysis in analytical chemistry. In this paper, the mechanism
of oxidation of L-Aspartic acid by dihydroxydiperiodatoargentate (III) is reported.
1.EXPERIMENTAL SECTION
1.1 Materials
All the reagents used were of A.R. grade. All solutions were prepared with doubly
distilled water. Solution of [Ag(OH)2(H2IO6)2]3-
(DPA) was prepared and standardized by the method reported earlier[5]. Its UV
spectrum was found to be consistent with that reported. The concentration of DPA was
derived by its absorption at l =351nm. Solution of DPA was always freshly prepared before use
with solution and doubly- distilled
water. The ionic strength m was maintained by adding KNO3 solution and the
pH value of the reaction mixture was regulated with KOH solution.
1.2 Apparatus and Kinetics Measurements
All kinetics measurements were carried out under pseudo-first order conditions.
Solution (2 mL) containing definite [Ag (III)], [OH-], [IO4-]
and ionic strength m and reductant solution (2mL) of appropriate concentration
were transferred separately to the upper and lower branch tubes of a type two-cell
reactor. After it was thermally equilibrated at desired temperature in thermobath (made in
Shanghai-Techcomp
Scientific Instrument Co., Ltd. ), the two
solutions were mixed well and immediately transferred to a 1 cm thick rectangular cell
quartz in a constant temperature cell-holder (¡À0.1ºC). The reaction process was monitored automatically by
recording the disappearance of Ag(III) with time (t) at 351 nm with a UV-8500
spectrophotometer (made in Shanghai-Techcomp Scientific Instrument Co., Ltd.). All
other species did not absorb significantly at this wavelength. Details of the
determinations are described elsewhere [6].
1.3 Product Analysis
Solution having known concentrations of [Ag (III)] and [OH-] was mixed with
an excess of L-Aspartic acid. The completion of the reaction was marked by the complete
discharge of Ag (III) color. After completion of the reaction, the oxidation product was
identified[7] as acid ketone.
2. RESULTS AND DISCUSSION
2.1Evaluation of Pseudo-First Order Rate Constants
Under the conditions of [Reductant]0>>[Ag(III)]0.,the
plots of ln(At-A¡Þ)
versus time are lines, indicating the reaction is first order with respect to
[Ag(III)], where At and A¡Þ are
the absorbance at time t and at infinite time respectively. The pseudo-first-order
rate constants kobs were calculated by the method of least squares (r¡Ý0.999). To calculate kobs generally 8-10 At
values within three times the half-life were used. kobs values were at
least averaged values of three independent experiments and reproducibility is within ¡À5%.
2.2 Rate Dependence on L-Aspartic acid
At fixed [Ag(III)], [OH-], [IO4-], ionic
strength m and temperature. k obs values increased
with the increase of [ASP] and the order in [ASP]was found to be 0< nap <1.
The plots of 1/kobs versus 1/ [ASP] are straight lines with a positive
intercept (Table 1) .
2.3 Rate Dependence on [OH-]
At constant [Ag(III)], [ASP], [IO4-], ionic strength m and temperature, kobs
values decreased rapidly with the increase in [OH-], and then increased slowly
with the increase in [OH-], The concentration of OH- was about 0.15
mol·L-1 at the turning point in which the rate was the slowest.(Fig 1)
2.4 Rate Dependence on [IO4-] and Ionic Strength m
At constant [Ag(III)], [ASP], [OH-], ionic strength m and
temperature, kobs values decreased slowly with the increase in [IO4-].
(Table2). The rate was increased by added KNO3 solution (Table3), which
is consistent with the common regulation of the kinetics[8].
Table 1103 kobs
(s-1) varying with different concentration of ASP at different temperatures
102C(mol·L-1) |
1.00 |
1.25 |
1.67 |
2.50 |
5.00 |
nap |
r |
a |
r1 |
T(K) |
¡¡ |
¡¡ |
¡¡ |
¡¡ |
¡¡ |
¡¡ |
¡¡ |
¡¡ |
¡¡ |
298.2 |
3.330 |
3.845 |
4.891 |
6.487 |
11.51 |
0.775 |
0.999 |
41.34 |
0.997 |
303.2 |
4.732 |
5.615 |
6.735 |
9.366 |
17.02 |
0.796 |
0.999 |
27.74 |
0.995 |
308.2 |
6.796 |
7.768 |
9.949 |
13.61 |
23.32 |
0.778 |
0.999 |
19.42 |
0.997 |
313.2 |
9.007 |
10.22 |
13.18 |
18.05 |
30.79 |
0.777 |
0.999 |
14.66 |
0.996 |
318.2 |
12.32 |
14.77 |
18.97 |
25.44 |
43.46 |
0.783 |
0.999 |
9.39 |
0.999 |
[Ag(III)]=7.924¡Á10-5mol·L-1; [IO4-] =2.00¡Á10-3mol·L-1; [OH-]=4.00¡Á10-2mol·L-1; m=0.192mol·L-1, nap
and r stand for the slope and relative coefficient, respectively, of the plot of lnkobs
vs lnC, a and r1 stand for intercept and relative coefficient, respectively, of the plot of 1/kobs
vs 1/[ASP].
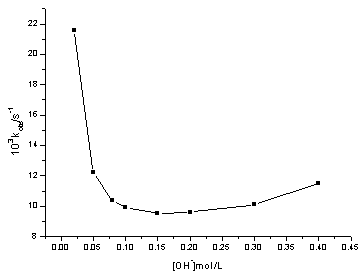
Fig.1 Plots of kobs vs [OH-]
,[Ag(III)]=7.924¡Á10-5mol·L-1; [IO4-]
=2.00¡Á10-3 mol·L-1; [ASP]=3.00¡Á10-2 mol·L-1; m=0.492 mol·L-1,
T=303.2K
Table 2 Rate
dependence on [IO4-]
10-3[IO4-](mol
·L-1) |
1.00 |
2.00 |
3.00 |
4.00 |
5.00 |
103kobs(s-1) |
10.39 |
9.818 |
9.717 |
9.583 |
9.565 |
[Ag(III)]=7.924¡Á10-5
mol·L-1;[OH-] =4.00¡Á10-2 mol·L-1;
[ASP]=3.00¡Á10-2 mol·L-1;m=0.135 mol·L-1,T=303.2K
Table 3 Rate dependence
on ionic strength m
m (mol·L-1) |
0.190 |
0.290 |
0.390 |
0.490 |
0.590 |
102 kobs(s-1) |
1.966 |
2.251 |
2.634 |
2.845 |
3.000 |
[ASP]=4.50¡Á10-2mol·L-1,
[Ag(III)]=7.924¡Á10-5mol·L-1, [OH-]=3.00¡Á10-2mol·L-1,
[IO4-]=2.00¡Á10-3mol·L-1, T=303.2K
3.DISCUSSION OF THE REACTION
MECHANISM
Based on the previous work[9,10,11], the main species of periodate are H2IO63-
and H3IO62-; the formula of Ag(III) periodate compiex may
be represented by [Ag(OH)2(H2IO6)2]5-.So
we proposed the reaction mechanism as below:
[Ag£¨OH-£©2(H2IO6)2]5+
£«OH- [Ag(OH-)2(HIO6)]3-
£«H2IO63-£«H2O
(1)
A
B
[-OOCCH2CHNH2COO-]2-£«H2O [-OOCCH2CHNH3£«COO-]£«OH-
(2)
[Ag£¨OH-£©2(HIO6)]3-£«[-OOCCH2CHNH3£«COO-]
complex
(3)
complex Ag(¢ñ) £«NH3£«-OOCH2COCOO- (4)
As the rate of the disappearance of [Ag(¢ó)]t is monitored and [Ag(¢ó)]t=[A]e£«[B]e £«[complex]e.
The reaction (4) is the rate-determining step:
-d[Ag(¢ó)]t/dt=k·[complex]e
(6)
kobs=
(7)
(8)
(9)
Equation (9) was given in the paper[10],from which we can
explain why kobs values decreased rapidly with the increase in [OH-]
up to 0.15 mol·L-1, but increased slowly with a further increase in [OH-],
The Equation (8) show that the order in ASP should be fractional order and 1/kobs
versus 1/[ASP] should be linear. The rate equations derived from the reaction
mechanisms are consistent with our experimental results. Based on intercepts of Table (1)
the rate-determining step constants (k) at different temperatures were evaluated,
then activation energy and the thermodynamic parameters ( 298.2K) were evaluated by the
method given earlier.[6](Table 4)
Table 4 Rate constants
(k) and activation parameters of the rate-determining step
Constants |
T(K) |
Activation
parameters (298.2K) |
298.2 |
303.2 |
308.2 |
313.2 |
318.2 |
Ea(KJ ·mol-1) |
D H#(KJ·mol-1) |
DS#(J·mol-1·K-1) |
102k(s-1) |
2.419 |
3.60 |
5.149 |
6.821 |
10.684 |
56.22 |
53.74 |
-95.74 |
Linear regression of lnk
vs 1/T gives intercept, a=18.95;slope, b=-6761.74;
relative coefficient, r=0.997
REFERENCES
[1] Kumar A. Vaishali. Ramamurthy P. Int. J. Chem. Kinet. 2000, 32: 286.
[2] Raviprasad T Sethuram B. Rao T N. Indian J. Chem, 1979, 18A: 40.
[3] Shan J H. Wei H Y. Wang L et al. Chemical Journal on Internet, 2001, 3 (11): 55
[4] Jaiswal P K,Yadava K L. Talanta. 1970, 17: 236.
[5] Balikungeri A, Pelletier M, Monnier D. Inorganica Chemica Acta, 1977, 22: 7
[6] Shan J H, Liu T Y, Acta Chimica Sinica (Huaxue xuebao) 1994, 52: 1140.
[7] Feigl F, Spot Tests in Organic Analysis, New York: Elsevier Publishing Co, 1956, 208.
[8] Jin J J. Kinetics Principie of Chemical Reaction in Liquid Phase, Shanghai: Science
Technique Press, 1984, 186.
[9] Aveston J. J. Chem Soc (A)., 1969, 273.
[10] Shan J H, Qian J Gao M Z et al. Chemical Journal on Internet, 2003, 5 (3): 21.
[11] Shan J H, Wei H Y, wang L et al. Journal of the Hebei Academy of Sciences (Hebeisheng
Kexueyuan Xuebao), 2001,18 (2): 93
¡¡
|