Supramolecular liquid
crystalline architectures by hierarchical self-assembly
ShenYongtao, Feng Xizeng, Chan QiLin,Peng Xiao
(The Key Laboratory of Bioactive Materials, Ministry of Education, Nankai
University, Tianjin 300071, China)
Received Mar. 15, 2005.
Abstract The structure of novel molecules
forming new types of liquid crystalline (LC) phases is of contemporary interest for
materials with useful application properties and also for the fundamental understanding of
soft-matter self-assembly. There are three main routes to tailor the self-assemble in LC
systems: these are the molecular shape, microsegregation effects and chirality. In
classical LC systems the shape of rigid units plays a dominating role for the organization
of the molecules, leading to nematic phases, layer structures (rodlike molecules; smectic
phases), and 2D arrangements of columns (disklike molecules; columnar phases). The advent
of supramolecular chemistry has provided chemists with a wealth of new possibilities to
synthesize materials in which the molecules are held together by relatively weak,
non-covalent interactions. It has become more and more attractive to assemble liquid
crystal with supramolecular interactions, and is named as supramolecular liquid crystal.
This review will focus on some recent discoveries in the field of spontaneous hierarchical
self-assembly of synthetic amphiphiles, disk-like molecules, rod-like, and dendrimer
building blocks into supramolecular liquid crystal.
Keyword supramolecular liquid crystal, disk-like, rod-like, dendrimer,
amphiphiles。
1 INTRODUCTION
In the past several decades liquid crystalline materials have received significant
attention. The combination of order and mobility in these systems leads to novel
functional materials which have had a great impact on recent development of mobile
information technologies. Although more than 100000 liquid crystalline compounds have been
synthesized thus far, their basic structures are quite similar. They represent either
anisometric molecules with a specific rodlike or disklike shape, or amphiphilic molecules, as well as oligomers, polymers, and dendrimers derived
from these fundamental structures. There are two main driving forces for self-organization
in such systems: (i) segregation of incompatible molecular parts into separate subspaces,
accompanied by aggregation of compatible or complimentary units, and (ii) minimization of
the free volume, which in the case of rigid molecules or supermolecular aggregates with a
rodlike or disklike shape leads to a parallel alignment of these anisometric units.
In the last decades of the 20th century, Pedersen, Cram and Lehn (Nobel
prize in 1987), initially working with crown ethers and cryptands, opened up new routes
toward constructing assemblies of molecules that are held by weak forces, e.g., hydrogen
bonding and van der Waals interactions. This area of research, called Supramolecular
Chemistry, has become increasingly popular in recent years. Now the Supramolecular liquid
crystal appears. The present review will further discuss these kinds of supramolecular
liquid crystal. Self-assembly and self-organization are key tools in supramolecular
chemistry and are now used by many research groups to build amazingly complex
architectures which are belong to the family of supramolecular liquid crystal.
Self-assemble not only plays an important role in supermocular systems but also serves as
a key driving force to assemble molecules to form liquid crystalline materials. A large
number of supramolecular liquid crystals have been built based on hydrogenbonding
interaction.
One strategy to obtain ordered ensembles of even higher dimensions is
by self-organizing these discrete aggregates into an extended lattice. Depending on the
fluidity of the self-assemble system one may distinguish two cases: hard self-assemble and
soft-assemble .[1]An example of hard self-organized matter is a crystal in
which there is little or no diffusion, while soft self-organized matter is represented by
a liquid crystal in which there is mobility in at least one direction. Soft matter, which
combines order and mobility on a microscopic and macroscopic level, is especially of great
interest in this respect, because such fluid self-organized systems are of fundamental
importance for technical applications as well as for the understanding of the basic
requirements for the formation of living matter. Supramolecular structures organized in a
two-dimensional (2D) lattice, are common in all types of organized soft matter [2].
They can be found as columnar mesophases (Col) built up by cylindrical polymolecular
aggregates formed by amphiphilic and dendritic molecules, block copolymers, and disklike
liquid crystals.
2. SUPRAMOLECULAR LIQUID CRYSTAL FORMED BY ROD-LIKE BUILDING BLOCKS
In recent years a wide variety of self-assembled, hierarchical, supramolecular
liquid crystal have been formed. Many of these make use of rod- like building blocks, held
together by a combination of hydrogen bonding, p-p stacking and van der Waals interactions. The group of Lehn has also
developed polymeric rigid rods that are formed by hydrogen bonding interactions between
complementary components containing two uracil and two 2,6-diacylaminopyridine groups.[3]
Apart from displaying a nematic thermotropic liquid-crystalline phase, the supramolecular
polymers also form a lyotropic liquid-crystalline phase in 1,1,2,2-tetrachloroethane
solution, as a result of molecular recognition phenomena between the rod-like subunits. In contrast to columnar mesophases of disklike molecules or columnar
aggregates of simple amphiphilic molecules, in which cylinders of one component are
surrounded by a fluid continuum , in the mesophase the space is subdivided into four
distinct sets of compartments: columns of the H-bonding networks, ribbons of aromatic
rods, columns containing the perfluorinated chains and regions with an enhanced
concentration of hydrocarbon chains. The results also show that quite simple low molecular
weight molecules, if appropriately designed, can lead to rather complex self-assmble
systems.
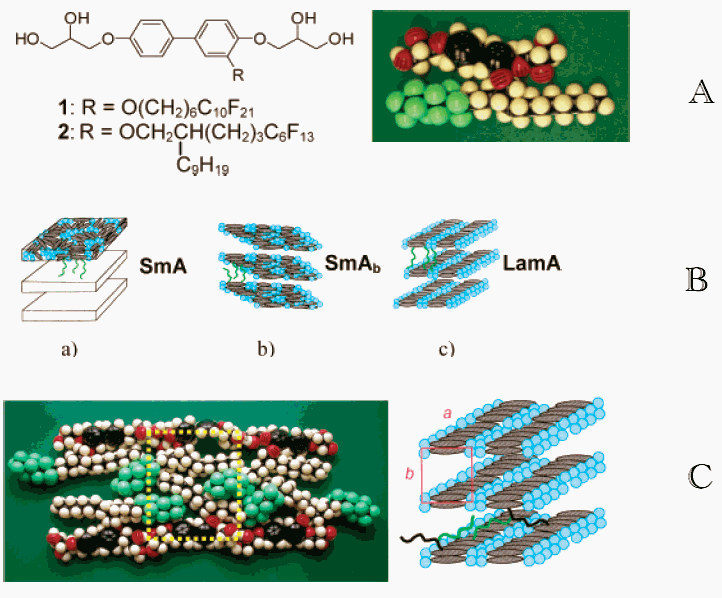
Figure 1 (A) Molecular structures of compounds 1 and 2 and CPK
model of a possible H-shaped conformation of compound 2. (B) The mesophases of compound 1: (a) SmA phase; (b) biaxial SmAb
phase with orientational correlation between adjacent 2D layers with nematic order; (c)
LamA phase: the H-bonding networks are segregated within the layers giving rise to a
periodicity within the aromatic sublayers, but adjacent layers are not positional
correlated. (C) Proposed model of the organization of compound 2 in the
columnar mesophase Colr.
Prehm, [4] has
synthesized the compounds such as 1 and related molecules, composed of a rodlike
rigid aromatic core, two polar hydrogen-bonding groups at the terminal ends and a
semiperfluorinated chain in a lateral position[5]were found to form novel types
of smectic liquid crystalline phases. In these mesophases the rodlike mesogenic segments
are organized parallel to the layer planes (Figure 1), which contrasts the
structures of all conventional smectic liquid crystalline phases where these units are
orthogonal or tilted with an angle unequal to 90°.
Cho and Lee [6,7 ] have shown that the rod building blocks
in coil -rod-coil tri block molecules based on an elongated rod self-assemble into
discrete cylinders that organize into a 3-D tetragonal superlattice above a certain coil
volume fraction. They have also demonstrated that
rod-coil diblock molecules based on an elongated rod can self-assemble into 3-D
honeycomblike supramolecular structure. [8 ] Their results imply that the
length of the rod building block has a strong influence on the shape of the supramolecular
structure. Consequently, systematic variation in the rod length would provide a novel
strategy to manipulate the supramolecular structure. They have synthesized rod-coil
triblock molecules with variation in the rod length at the identical rod to coil volume
ratio and investigated their structural behavior. The results described demonstrate that,
as the chain length of the molecule increases at the fixed rod to coil volume ratio, the
self-assembled 3-D structure changes significantly from organized rod-bundles in a coil
matrix (tetragonal structure) to organized coil perforations in rod layers (honeycomb
structure)(Figure 2).
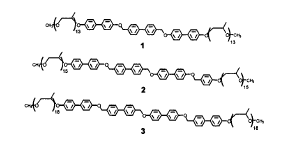 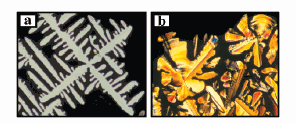
Figure 2 Compound 1 ,2,3
.Representative optical polarized micrographs (100x) of the textures exhibited by (a) the
body centered tetragonal mesophase of 2 at156 oC and (b) the honeycomb
mesophase of 3 at 195 oC on the cooling scan.
LC molecules with a bent
molecular shape, so-called banana-shaped liquid crystals, have attracted special
attention, because such materials organize into fluid phases with polar order and
supramolecular chirality[9], properties which are of current
interest in different areas of science. The banana-shaped liquid crystals are
unusual forms of rod-like compounds (Figure 3).
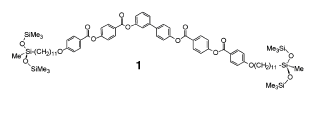
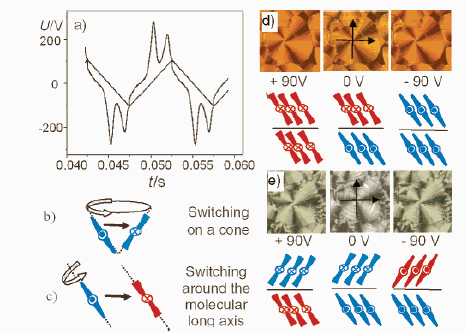
Figure 3 A kind of the
banana-shaped compound 1. (a) Switching current response curve obtained by applying a
triangular-wave voltage at 116 °C; the two current peaks within one half-period of the
applied voltage indicate an antiferroelectric (AF) switching. (b) Switching on a cone
reverses only the layer polarity. (c) Switching around the molecular long axis reverses
both polarity and layer chirality (chirality sense is indicated by red/blue).(d, e) Due to
the switching around the long axes, the extinction crosses of the circular domains do not
change their position by reversing the sign of the applied voltage; arrows give the
positions of the polarizer and analyzer.(d) ColAF phase at 130 °C. (e) SmCAPA
phase at 115 °C.
The polar order results
from the directed organization of these molecules with the bend angles of adjacent
molecules pointing into the same direction. Chirality arises due to the tilted
organization of these nonchiral molecules in polar layers. Polar direction, tilt
direction, and the layer normal define either a right-handed or a left-handed system,
changing either polarization direction or tilt direction changes the chirality sense
of the layer[10]. Such soft matter systems, capable of spontaneously generating
polar and chiral superstructures, which can be switched by external fields, could lead to
novel functional materials. Keith[11] synthesized a kind of bent core
molecules, related to 1 (figure 3). Attaching one oligosiloxane unit to such
molecules leads to a transition from antiferroelectric switching to ferroelectric
switching liquid crystals. It was the first bent core molecules containing oligosiloxane
units at both ends, and it was found that this gives rise to (i) the formation of
modulated smectic phases in which the molecules adopt an antiferroelectric polar order,
(ii) extremely large tilt angles, and, most important, (iii) a field-induced switching of
the molecules by collective rotation around the molecular long axes, a process which
switches the chirality sense of the layers.
Rod building blocks can generate organized objects of nanoscale
dimensions through the combination of shape complementarity and the repulsive interactions
of rigid and flexible parts. Lee and his co-workers[12] have demonstrated that
the self-assembled structure based on rod building blocks can be manipulated through the
attachment of flexible parts of different lengths to their ends. Depending on the relative
length of the flexible segments, these blocks self-assemble into infinitely long cylinders
or dissimilar cylinders that organize into two-dimensional, hexagonal or
three-dimensional, tetragonal superlattices. Honeycomb supramolecular structures assembled
from elongated rods and able to self-assemble into a three-dimensional hexagonal
superlattice[13]. Lee et al also have reported an especial rod-like
molecular "dumbbell-shaped" molecules
consisting of three biphenyls connected through vinyl linkages as a conjugated rod segment
and aliphatic polyether dendritic wedges with different crosssections were synthesized and
characterized (Figure 4).
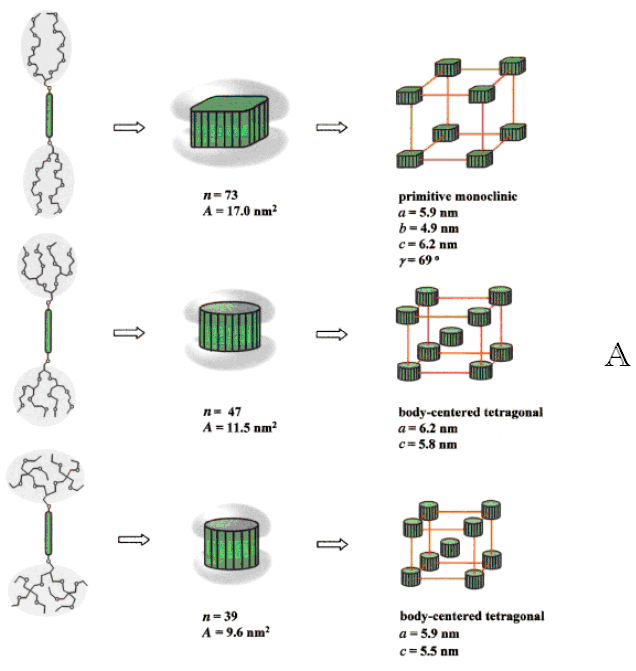
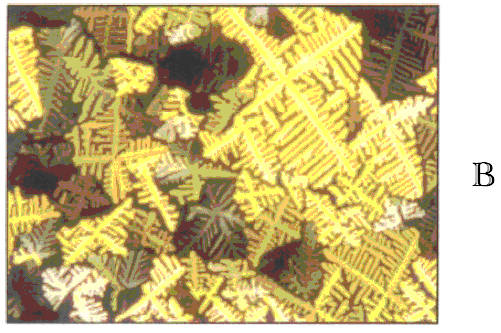
Figure 4 (A) Structural analysis of the supramolecular
bundles assembled from molecular dumbbells 1--3; n is the number of molecules per
aggregate and A is the cross sectional area of the rod-bundle. (B) Representative optical
polarized micrograph of texture exhibited by a body-centered tetragonal micellar mesophase
of 1 at the transition from the isotropic liquid state at 220 oC.
The
molecular dumbbells self-assemble into discrete bundles
that organize into three-dimensional superlattices. Molecule 1 (figure 4), based on
a dibranched dendritic wedge, organizes into primitive monoclinic-crystal and
body-centered, tetragonal liquid crystalline structures, while molecules 2 and 3
(figure 4) based on tetra- and hexabranched dendritic wedges, respectively, form only
body-centered, tetragonal liquid crystalline structures. X-ray diffraction experiments and
density measurements showed that the rod-bundle cross-sectional area decreases with
increasing cross-section of the dendritic wedges. The influences of supramolecular
structure on the bulk-state optical properties were investigated by measuring the
absorption of UV/V and steady state fluorescence spectroscopies. As the cross-section of
the dendritic wedge of the molecule increases, the absorption and emission maxima shift to
higher energy. This can be attributed to a quantum size effect of the three-dimensionally
confined nanostructure. Spectroscopic studies of these molecules demonstrate that the size
of the optically active rod bundle has an influence on the photophysical properties of the
conjugated rods, and consequently their approach to controlling the size of the
nanostructure by synthesis provides a strategy for controlling supramolecular material
characteristics.
3.SUPRAMOLECULAR
LIQUID CRYSTAL FORMED BY DISK-LIKE AND DENDRIMER BUILDING
BLOCKS
This approach was elegantly demonstrated by Zimmerman and Lehn, who constructed
disk-like mesogens by the self-assembly of lactim–lactam
trimers, which were then found to stack together yielding columnar, discotic liquid
crystalline phases [14] The disubstituted phthalhydrazide building blocks thus
form the recognition motif for the induced generation of large supramolecular
architectures. While hierarchical self-assembly processes in the liquid crystalline state
are generally governed by directional intermolecular interactions such as hydrogen bonding
and p-p stacking, such
processes in solution become more complicated and often less predictable due to the
influence of hydrophobic or solvophobic effects.
Marcos and his co-workers[15] have reported the liquid crystalline
properties of some poly(amidoamine) (PAMAM) dendrimers functionalized in their terminal
groups by one-chain promesogenic units. In these compounds the weak interactions between
the mesogenic units make the molecules adopt a cylindrical geometry, which resembles a
heavy thick rod-like structure that induces smectic phases. They present the results
obtained for a series of homologous PAMAM dendrimers that carry in the terminal
promesogenic units with two decyloxy chains in the 3- and 4-positions of the peripheral
aromatic ring. This change modifies the relationship between the hard part of the
dendrimer and the soft part, corresponding to the PAMAM central structure and the alkoxy-
terminal chains, respectively. As a consequence of this additional terminal chain, the
molecular interactions are modified and the molecules adopt another conformation that
induces columnar mesomorphism (Figure 5).
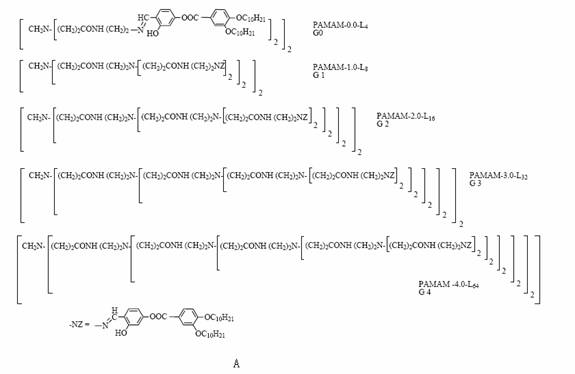
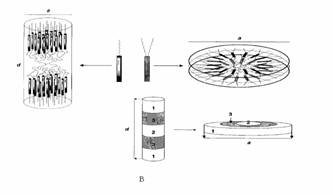
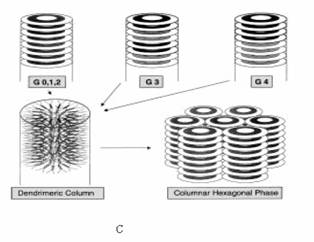
Figure 5 (A) Structure of
the five generation dendrimers. (B) Giant rods versus giant discs. Soft parts of
the dendrimer (white areas): 1) terminal alkyl chains, 2) central PAMAM groups; hard parts
of the dendrimer (gray areas): 3) promesogenic units. (C) Supramolecular
organization of the dendrimers within the columnar mesophase.
Percec and his coworkers[16]
have reported they had synthesized the benzyl ether based self-assembling
monodendrons containing benzo[15]crown-5 at their focal point. These dendritic building
blocks self-assemble and subsequently self-organize either spontaneously or after
complexation with NaOTf into smectic B, smectic A, p6mm hexagonal columnar (Fh) and Pm n cubic lattices.
The dependence between the shape of the monodendron, the shape of the supramolecular
dendrimer, the symmetry of the supramolecular lattice obtained by its spontaneous
self-assembly and self-organization and the influence of complexation with NaOTf on the
stability of various supramolecular lattices were elucidated by retrostructural analysis
of the lattices generated from supramolecular dendrimers. A very delicate dependence
between the shape of the dendritic crown ether and the stability of its supramolecular
lattice obtained by complexation with NaOTf was demonstrated and mechanisms of
complexation mediated self-assembly were suggested. These mechanisms many help to
elaborate novel ionactive supramolecular concepts. (Figure 6)
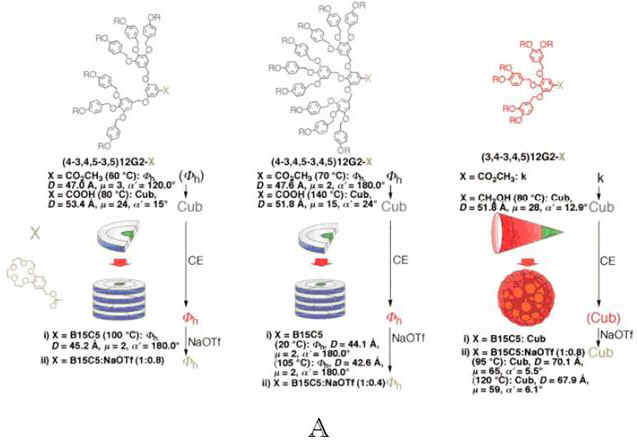
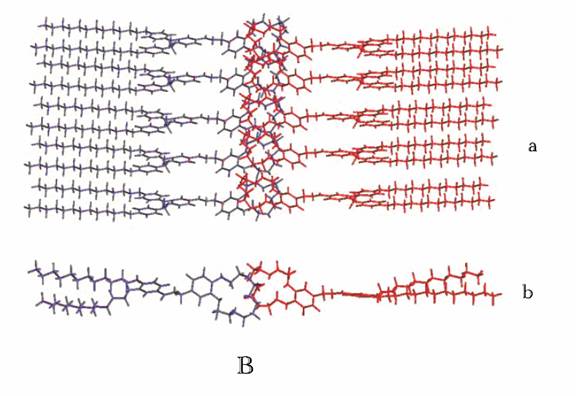
Figure 6 (A) Retrostructural
analysis of supramolecular dendrimers self-assembled from dendritic crown ethers (CE) (B)
Molecular models of the smectic layers of a) smectic A (SA) and b) smectic B (SB) phases.
Liquid crystals represent
a unique class of self-organizing systems, which although found in many day-to-day
practical material applications, such as displays, are also intimately entwined with
living processes. Saez and Goodby[17] described a new concept for the design of
self-assembling functional liquid crystals as segmented or "Janus" liquid-crystalline
supermolecular materials in the form of structures that contain two different types of
mesogenic units, which favour different types of mesophase structure, grafted onto the
same star-shaped scaffold to create supermolecules that contain different hemispheres. The
materials exhibit chiral nematic and chiral smectic C phases.
The basic design concept (Figure 7) consists of creating a star-shaped
scaffold that contains two chemically different halves, capable of being independently
manipulated, to which appropriately functionalized mesogenic sub-units may be covalently
attached. The type of mesogen, the geometry of the attachment of the mesogens to the
central scaffold, and the nature of the connecting group were explored as tools form
creating supermolecular systems with different halves. The use of aliphatic spacers
between the mesogenic moieties and the scaffold follows the same concept as in traditional
side-chain liquid-crystalline polymers, that is, to decouple the motions of the mesogenic
units from the scaffold thereby allowing their ordering in the creation of a mesophase. In
this case they placed particular emphasis in studying the effect of segregation of chiral
groups within the molecule on molecular recognition processes that ultimately lead to
selective mesophase formation.
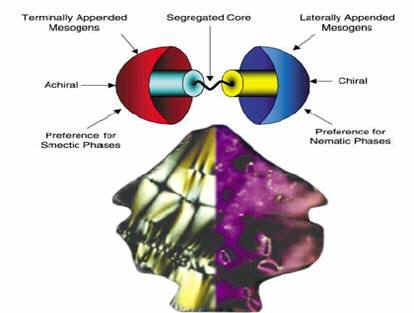
Figure 7 Design concepts for "Janus"supermolecular
liquid crystals with different hemispherical architectures.
Percec[18] has
reported a new kind of compound. The synthesis and structural analysis of a polymer
containing twindendritic benzamide side-groups (i.e.
poly{N-[3,4-bis(n-dodecan-1-yloxy)-5-(1-methacryloyl-n-undecan-1-yloxy)-phenyl]-3,4,5-tris(n-dodecan-1-yloxy)-benzamide})
are described. The disc-like side groups of this polymer self-assemble into supramolecular
cylindrical dendrimers through hydrogen bonding along the column long axis, creating a
novel architecture consisting of a polymer chain(s) coated with a three-cylindrical bundle
supramolecular dendrimer. This polymer self-organizes in a thermotropic nematic liquid
crystalline (LC) phase. The low molar mass twin dendritic benzamide, which has a similar
structure to that of the polymer side groups, self-assembles into supramolecular
cylindrical dendrimers, which self-organize on a two dimensional hexagonal columnar (Fh) LC lattice. Co-assembly of the
polymer produces a novel two dimensional (Fh) LC superlattice. The mechanism responsible for this co-assembly
provides access to libraries of functional two-dimension Fh superlattices. Hydrogen bonding along the center of the
supramolecular column is an important structural parameter that determines the
self-assembly and co-assembly of these twin-dendritic building blocks. It demonstrated the
potential use of this new and simple concept for the elaboration of other novel LC
superlattices from twindendritic building blocks. [19] (Figure 8)
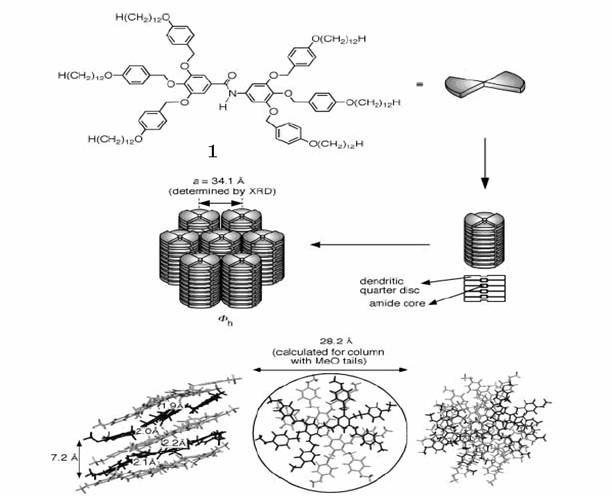
Figure 8 Coassembly of the
hexagonal columnar liquid crystalline superlattice from 1.
Ishi-i [20]and his co-workers have
reported unique and unusual formations of columnar liquid crystals and organogels by
self-assembling discal molecules, which are composed of an aromatic hexaazatriphenylene
(HAT) core and six flexible aromatic side chains. The aromatic side chains with terminal
flexible groups make up soft regions that cooperatively stabilize the liquid crystal and
organogel supramolecular structures together with the hard regions of the
hexaazatriphenylene core.
Yelamaggad [21]has discovered a unique class of discal LCs derived
from symmetries of tris(N-salicylideneanilines) existing exclusively in
their C3h and Cs ketoenamine tautomeric forms. These compounds
display Col LC phases in which the proton and electron interact with each other through
the H-bonding environment. Remarkably, the core-core separation is quite small, making
them as the promising materials for many proposed electronic devices.
4 SUPRAMOLECULAR LIQUID CRYSTAL FORMED BY AMPHIPHILES BUILDING BLOCKS
The study of amphiphilic molecules and the
architectures they form them is an area of science that dates back more than a hundred
years, and was initially primarily focused on soaps, fats and oils. Without doubt the most
important family of amphiphiles is that of the phospholipids, which self-assemble to form
the walls of living cells. [22] As a consequence of their industrial
importance, numerous investigations have been carried out to unravel the relationship
between the supramolecular architectures formed by the amphiphiles and their molecular
structure, e.g., the determination of the ratio between the hydrophilic and hydrophobic
components in the amphiphile, culminating in the shape–structure rules defined by Israelachvili. [23]
In the nature, self-assembled architectures formed by
amphiphilic molecules are often hierarchically organized and composed of smaller units
(micelles, bilayers). It has been observed that in many cases the final micrometer-sized
structures are the result of a higher level aggregation process involving these smaller
units. Synthetic amphiphiles, for example gluconamides[24], can also display a
similar hierarchical self-assembly process. When dispersed in water, these molecules first
aggregate into micellar strands, which in subsequent steps further assemble to yield
multi-helical superstructures, and even "molecular braids" consisting of inter-twined helical strands.
Felekis and his co-workers[25] have reported the synthesis
and characterization of hydrogenbonded dendrimeric liquid crystals.(Figure 9). For
preparing this type of hydrogen-bonded complexes a two-stage strategy can be followed:
Initially, at the external groups of hyperbranched polymers recognizable moieties are
attached, which interact, at a second stage, through hydrogen bonding with mesogenic
molecules bearing complementary moieties. The resulting hydrogen bonded materials can
potentially exhibit liquid crystalline character. In their study, pyridinyl moieties were
introduced at the external surface of a polyglycerol (hyperbranched polyether polyol, Mn=
5000, PG) through esterification of its hydroxy groups with isonicotinoyl chloride
hydrochloride. Pyridinyl groups were subsequently interacted, through hydrogen bonding
with succinic acid monocholesteryl ester, Chol-I, pentanedioic acid monocholesteryl ester,
Chol-II, or succinic acid mono(5-cholesteryloxypentyl) ester, Chol-III. In this manner,
cholesteryl moieties were noncovalently attached at the external surface of the
hyperbranched polymer, and the role of the spacers in modifying the liquid crystalline
character of these supramolecular complexes was investigated. The liquid crystalline
character of the hydrogen-bonded complexes was identified with polarized optical
microscopy and differential scanning calorimetry and established by X-ray diffraction.
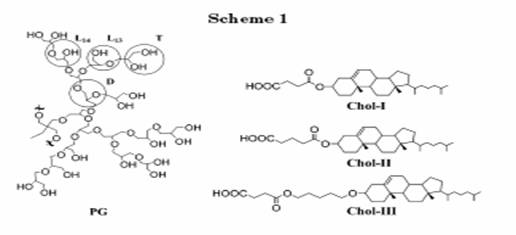
Figure 9 amphiphiles
synthesized by T. Felekis.
Milkereit [26]
has reported a new kind of amphiphile. Two alkyl glycosides with the same type of
disaccharide headgroups (melibiose) and different methyl-branched alkyl chains, short
chiral [(2R,4R,6R,8R)-2,4,6,8-tetramethyldecyl, extracted from
an animal source and long nonchiral
(3,7,11,15-tetramethylhexadecyl, from a plant source), were synthesized. The
supramolecular aggregate structure formed in dilute
solutions was investigated by small-angle neutron scattering and surface tension measurements. The lyotropic phase diagram was studied by
differential scanning calorimetry and water
penetration scans. The thermotropic phase behavior was investigated by polarizing
microscopy. The compounds showed unusual phase
behavior: (i) The liquid-crystalline polymorphism is reduced to only form smectic A phases in the pure state. (ii) The compound with the
longer nonchiral alkyl chain is more soluble in water than the one with the shorter chiral chain. (iii) For the long-chain compound the short-chain compound forms large disklike/bilayer aggregates. The method of methylation of the chain
controls the self-assembly and can explain different
biological functions for either plants (variable temperature) or animals (constant
temperature) (Figure 10 A).
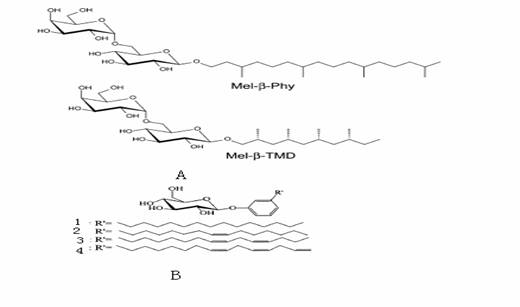
Figure10 amphiphiles synthesized by
Go1tz Milkereit and Jong Hwa Jung.
Jong [27] has
synthesized analogous compound components 1-4 with unsaturated bonds was achieved
because the diene and triene derivatives 3 and 4 are existing as liquid
crystals at room temperature.(Figure 10 B)
Recently there is a new kind of amphiphilic appears. Many people call
it Bolaamphiphilic molecule. Bolaamphiphilic molecules contain a hydrophobic skeleton (e.g., one, two, or three alkyl chains, a steroid, or a porphyrin) and two water-soluble groups on both ends. Bolaamphiphiles are related to and often combined with “edge
amphiphiles”, where one flank of a hydrophobic core carries hydrophilic groups whereas the other edge is hydrophobic (Figure 11)
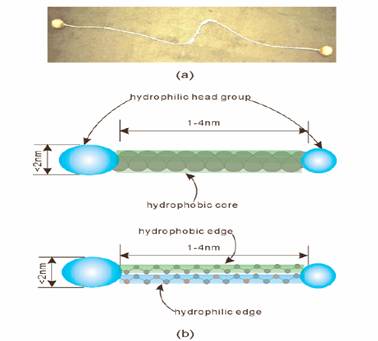
Figure 11 (a) Photograph of
an argentine bola with leather balls, that resemble bolaamphiphile ; (b) schematic
drawings of a bolaamphiphile (abbreviation, bola). Green coloring indicates hydrophobic
parts; blue means hydrophilic. Red will be used for conjugated systems, usually dyes, as
well as for oxygenatoms in structural formula.
Mickaëlle Brard and his co-workers[28] have reported a
Bolaamphiphile. They have synthesized unsymmetrical archaeal tetraether glycolipid
analogues 1-2 incorporating a 1,3-disubstituted cyclopentane ring into the
bridging chain. The cyclopentane has been introduced with a totally controlled cis
configuration, either into the middle of the aliphatic chain or at three methylene groups
from the glycerol unit linked to the bulkier disaccharide residue. Freeze-fracture and
cryotransmission electron microscopy experiments clearly demonstrated unprecedented
glycolipid supramolecular organizations involving two-by-two monolayer associations
coupled with interconnection and fusion phenomena. Furthermore, a significant difference
in the hydration properties and in the lyotropic liquid crystalline behavior of bipolar
lipids 1-2 was found depending on the position of the cyclopentane
residue.(see figure 12)
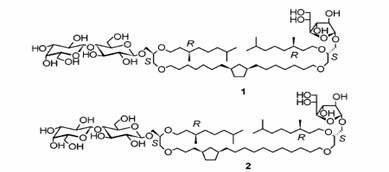
Figure 12 Synthetic unsymmetrical
bolaamphiphile 1-2.
5 CONCLUSION AND OUTLOOK
Following the historical order from liquid crystalline molecules to
macromolecule liquid crystal to supramolecule liquid crystal, the next challenge will be
the design of liquid crystal combined with matter of life. These are fluid anisotropic
phases in which building blocks self-assemble such that they stack one on top of the other
to form Col structures and are promising for potential applications[29] such as
one-dimensional conductors, [30] photoconductors, [31] molecular
wires and fibers, [32] light emitting diodes, [33] and photovoltaic
cells. [34] A synergistic approach in which the interaction between physics,
chemistry, and biology plays a pivotal role offers the best chance of success for
mastering molecular matter in the future.
REFERENCES
[1] Tsai C J, Ma B J, Kumar S et al. Rev. Biochem. Mol., 2001, 36: 399.
[2] de Gennes P G. Angew. Chem., Int. Ed. Engl. 1992, 31: 842.
[3] Kotera M, Lehn J M, Vigneron J P. J. Chem. Soc., Chem. Commun., 1994, 197.
[4] Prehm M, Diele S, Das M K et al. J. Am. Chem. Soc. 2003, 125: 614.
[5] Ko¨lbel M, Beyersdorff T, Cheng X H et al. J. Am. Chem. Soc. 2001, 123: 6809.
[6] Cho B K, Lee M. J. Am. Chem. Soc. 2001, 123: 9677.
[7] Lee M, Cho B K, Jang Y G et al. J. Am. Chem. Soc. 2000, 122: 7449.
[8] Lee M, Cho B K, Ihn K J et al. J. Am. Chem. Soc. 2001, 123: 4647.
[9] Niori T, Sekine F, Watanabe J et al. J. Mater.Chem. 1996, 6: 1231.
[10] Link D R, Natale G, Shao R et al. Science 1997, 278: 1924.
[11] Keith C, Reddy R A, Baumeister U et al. J. Am. Chem. Soc. 2004, 126: 14312.
[12] Lee M, Jeong Y S, Cho B K et al. Chem. Eur. J. 2002, 8 (4): 876.
[13] Lee M, Cho B K, Ihn K J et al. J. Am. Chem. Soc. 2001, 123: 4647.
[14] Sua'rez M, Lehn J M, Zimmerman S C et al. J. Am.
Chem. Soc., 1998, 120: 9526.
[15] Marcos M, Gime?nez R, Serrano J L et al. Chem. Eur. J. 2001, 7(5):1006
[16] Percec V, Cho W D, Ungar G et al. Chem. Eur. J. 2002, 8 (9):2011.
[17] Saez I M, Goodby J W. Chem. Eur. J. 2003, 9: 4869.
[18] Percec V, Ahn C H, Tushar K et al. Chem. Eur. J. 1999, 5 (3): 1070.
[19] Percec V, Bera T K, Glodde M et al. Chem. Eur. J. 2003, 9 (4): 921.
[20] Tsutomu I, Tomoyuki H, Murakami K et al. Langmuir 2005, 21: 1261.
[21] Yelamaggad C V, Achalkumar A S, Rao D S et al. J. Am. Chem. Soc. 2004, 126: 6506.
[22] Feiters M C, NolteR J M. in Advances in Supramolecular Chemistry, vol. 6, ed. G. W.
Gokel, Jai Press, Stanford, CT, USA, 2000, pp. 41—156.
[23] Israelachvili J. Intermolecular & Surface Forces, 2nd edn., Academic Press,
London, 1992, part III.
[24] Fuhrhop J H. Chem. Rev., 1993, 93: 1565.
[25] Felekis T, Tziveleka L, Tsiourvas D et al. Macromolecules, 2005, 38(5):
1705
[26] Milkereit G, Garamus V M, Yamashita J et al. J. Phys. Chem. B., 2005, 109: 1599.
[27] Jong H J, John G, Yoshida K et al. J. Am. Chem. Soc. 2002, 124: 10674.
[28] Brard M, Richter W, Benvegnu T et al. J. Am. Chem. Soc. 2004,
126:10003.
[29] Bushby R J, Lozman O R. Curr. Opin. Colloid Interface Sci., 2002, 7:343.
[30] Chandrasekhar S, Balagurusamy V S K. Proc. R. Soc. London, Ser. A, 2002, 458: 3.
[31] Adam D, Schuhamcher P, Simmerer J et al. Nature 1994, 371: 141.
[32] Osburn E J, Schmidt A, Chau L K et al. Adv. Mater. 1996, 8: 926.
[33] Christ T, Glusen B, Greiner A et al. Adv. Mater. 1997, 9: 48.
[34] Schmidt-Mende L, Fechtenkotter A, Mullen K et al. Science, 2001, 293:
1119.
逐级自组装形成的超分子液晶
沈永涛,冯喜增,产启林,彭萧
(南开大学活性材料教育部重点实验室,天津300071,中国)
摘要 设计新颖分子构筑新型液晶材料的研究备受关注,特别是新材料的制备与应用,有利于深入理解自组装软物质形成的机制。自组装液晶体系的制备主要通过分子的形状、微偏析效应和手性三种特性方法。在传统液晶体系中分子的形状起了关键作用,形成向列相、层结构(如棒型分子形成近晶相)、二维柱型结构(如碟型分子形成圆柱相)液晶。超分子化学的出现为化学家提供了许多合成新型材料的可能性,这些材料中的分子由一些较弱的力即非共价键结合在一起。将超分子与液晶相结合越来越引起人们的兴趣,我们称之为超分子液晶。这篇综述主要着眼于利用双亲分子、碟型分子、棒型分子和枝状分子逐级自组装超分子液晶方面的最新进展。
关键词 超分子液晶,碟型分子,棒型分子,枝状分子,双亲分子。
|