Preparation and
characterization of porous chitosan membranes and the localization of the activity of
urease immobilized on it by SEM and X-ray microanalysis
Ma Xiaolia,b, Yao Zihua* a, Shi
Daganga
(a College of Chemistry and Environmental Science, Hebei University, Baoding,
071002; b Hebei Medical College for Continuing Education, Baoding 071002,
China)
Abstract Porous chitosan membranes were
prepared using dibutyl phthalate as porogen. The structure of
the surface and the cross section of porous chitosan membranes were examined by SEM. The
distribution of pore size and mean pore diameter were analyzed by particle size analysis
system of SEM. It shows that the porous chitosan membranes possess controlled pore sizes,
thoroughfare pores, and the pore diameters ranged from 0.3mm to 70mm and mean pore diameters ranged from 0.560mm to 15.542mm. Then urease was immobilized on porous chitosan membrane and the
localization of the activity of urease immobilized on membrane was studied by X-ray
microanalysis. BaCl2 and urea were selected as capture and substrate
respectively. The substrate was hydrolyzed by immobilized urease to produce NH3
and CO2 in Tris-HCl buffer (pH7.0), and the latter was captured by BaCl2
to form BaCO3. The precipitation deposited on the active site of immobilized
urease. Investigation shows that porous chitosan membranes possess larger surface area and
more amount of urease immobilized than on non-porous chitosan membrane, the activity of
urease immobilized on porous chitosan membrane is 17.22 U/cm2·min,
and 5 times as large as that of urease immobilized on non-porous chitosan membrane (3.6
U/cm2·min). The distribution of activated
enzyme was uniform on the surface and the inside of porous chitosan membrane.
Keywords Porous chitosan membrane; SEM; X-ray microanalysis; Immobilized urease.
1 INTRODUTION
Chitosan, an inexpensive, inert, hydrophilic, biocompatible linear polymer, derived
from the exoskeletons of insects and shells of crustaceans, is the second most abundant
natural polysaccharide after cellulose. The chemical structure of chitosan is b-(1,4)-2-amino-2-deoxy-D-glucose which
is obtained by partial deacetylating chitin. As they are non-toxic, biodegradable and
biocompatible, chitin and chitosan have been used in a wide variety of biomedical
application, such as enzyme carriers, wound dressing, drug delivery carriers, tissue
engineering, bone healing materials, chromatographic media, and so on. In many cases of
such applications, it is necessary to have a porous structure.
Many works have been reported to obtain chitin or chitosan matrix with
porous structure. One of the most tried methods is the phase inversion technique followed
by various lyophilization strategies (i.e. Polymer solution is cast and immersed in a
coagulation bath followed by lyophilization). The porous chitin or chitosan matrix
prepared by this method have been used as tissue engineering to seed cell [1-3],
wound dressing [4,5] and drug delivery carriers [6-8].
Chow and Khor developed a novel method called "internal bubbling
process" (IBP) to give open and large-pored
chitin matrixes [9]. Essentially, IBP was achieved by first adding CaCO3 into
a chitin solution to form a stable suspension that was cast into a mold to give a CaCO3-chitin
gel. The CaCO3-chitin gel was next submerged in a 1N HCl solution, initiating a
chemical reaction that generated CaCl2 and gaseous CO2 creating the
IBP throughout the chitin gel continuously from within the bulk to the surface. The result
was an open structure that upon air-drying gave a highly porous chitin matrix of uniform
large pore sizes ranging from 100 to 1000mm depending on the amount of CaCO3 added into the chitin
solution. The CaCl2 was readily removed by washing the chitin gel with water in
a pre-drying step that left no calcium-based residue.
Zeng et al. [10-12] prepared macroporous chitin and chitosan
membranes with controlled porosities and very good mechanical properties, using silica
particles as porogen. This method consists of casting a suspension of silica particles of
selected size in an acidic chitosan solution, removing the solvent by evaporation and
selectively dissolving the silica particles in an alkaline solution (chitosan is soluble
in acidic, but insoluble in alkaline solutions) afterwards. The porous chitosan membranes
obtained by this method showed efficient for proteins and enzymes purification.
In another paper, Steenkamp[13] manufactured a composite
alumina/chitosan membrane to remove Copper (II) from polluted water. The porous alumina
support was manufactured with a centrifugal casting technique. Pore radii varied between
45 and 100nm. The support tubes were provided with a chitosan coating of about 15mm thickness. The porosity of the
coating was controlled with a phase inversion method using silica as a porogen. Through
these composite membranes a 50mg/L Cu2+ solution was decreased to a
concentration level below 1mg/L.
Microporous chitosan membranes can also be prepared using PEG as
porogen [14]. This was achieved by first adding polyvinyl pyrrolidone (PVP) or
polyethylene glycol (PEG) to blend with chitosan, and removing the solvent by evaporation,
then selectively dissolving PEG or PVP in hot water. The pore structure induced by this
method is controlled by the compatibility of the chitosan and the counterpart polymers. No
pore structure was induced in the case of chitosan/PVP, because of their molecule level
miscibility and strong interaction. Highly porous structure was induced in the case of
chitosan/PEG due to their poor compatibility and multiphase structure.
In this article, we developed a novel method to prepare porous chitosan
membranes, using dibutyl phthalate as porogen. The porous chitosan membranes possess
controlled pore sizes, thoroughfare pores. The membranes were prepared by casting acidic
chitosan solution with added dibutyl phthalate, removing the solvent by evaporation, then
dissolving dibutyl phthalate by acetone. The porous structures of surface and cross
section were examined by SEM analysis. The distribution of pore size and mean pore
diameters were analyzed by particle size analysis system of SEM. These porous chitosan
membranes would be used as enzyme carriers, wound dressing, drug delivery carriers and
tissue engineering.
The presence of hydroxyl and amino groups facilitates the
immobilization of enzymes on chitosan or chitin by both adsorption and covalent linkage. Chitosan can be used in the form of powder, gel,
beads, membranes and also as transparent film. Chitosan membranes have been used for
immobilization of urease [15, 16], catalase [17], lipases [18,
19], tyrosinase [20] as enzyme sensors or reactors.
Here, urease was immobilized on porous chitosan membrane. The
localization of the activity of immobilized urease on it was studied by X ray
microanalysis.
2 EXPERIMENTAL
2.1 Materials and apparatus
Chitosan was 90%deacetylated. Urease, 71.1 unit/mg, was a commercial product from
Worthington biochemical corporation. Tris and glutaraldehyde were biochemical reagents and
all the other reagents used were of analytical grade.
KYKY—1000B scanning electron
microscopy (SEM) with a Thermo NORAN-Vantage X-ray energy dispersive spectrometer (EDS)was used in this
investigation.
2.2 Preparation of porous chitosan membranes
2.5 grams of chitosan was dissolved in 100ml of 2% aqueous acetic acid solution overnight.
Then dibutyl phthalate was added to this solution, followed by mixing with ultrasonic wave
in order to homogenige them. The solution was cast onto a piece of glass and the solvent
was allowed to evaporate. The membrane was neutralized with 1% NaOH solution for 2 h and
washed with redistilled water repeatedly. The membrane was immersed in acetone that
dissolved dibutyl phthalate to generate a porous chitosan membrane. The porous chitosan
membranes with different pore size were prepared by this method.
2.3 Scanning electron microscopy (SEM)
The structure and morphology of porous chitosan membranes were examined by using scanning
electron microscopy (SEM). Samples were randomly chosen from different batches of
membrane. The SEM photographs of the samples' surface
were obtained by the samples that were coated with a conductive layer (400Å) of
sputtered gold. The SEM photographs of the samples' cross-section
were obtained by the samples that were first embedded into epoxy resin and fractured
cryogenically in liquid nitrogen, then were coated with a conductive layer (400Å) of
sputtered gold. After that, the samples were imaged and photographed by employing a
scanning electron microscope (KYKY—1000B SEM) at 25
kV accelerating voltage. The distribution of pore size and mean pore diameters were
determined by particle size analysis system of SEM.
2.4 Immobilization of urease on porous chitosan membrane
Urease was immobilized according to the following general procedure[21]: the
membrane was immerged in an urease solution containing 2 mg of urease per ml of a pH 5.6
phosphate buffer for 1 h at room temperature with occasional stirring, and left overnight
at 4ºC. The next day, the membranes was washed with redistilled water
followed by a pH 7.0 phosphate buffer solution, then treated with a 0.01% glutaraldehyde
solution for 60 min and washed with redistilled water until the washings were free of
glutaraldehyde. Finally immobilized urease was kept in a pH7.0 phosphate buffer solution.
2.5 Determination of the activity of the immobilized urease
The activity of immobilized urease was determined by using the Weatherburn method [22].
2 ml phosphate buffer (pH7.0) containing 1% urea and 1 mM EDTA was kept in a test tube and
a piece of membrane immobilized urease was introduced in and incubated in a 37ºC water bath for
30 min. Then 10ul of above solution was mixed with 1 ml reagent A (5% phenol-0.025% sodium
nitroprusside) and 1 ml reagent B (2.5% sodium hydroxide-0.21% sodium hypochlorite) and
the total volume was made up to 10 ml with redistilled water. After color developing at 37ºC for 20 min,
the absorbance was read at 625 nm using a spectrophotometer. The amount of ammonia
liberated was calculated by comparing the absorbance with a standard curve for ammonium
sulphate. One unit of enzyme activity was defined as the amount of enzyme that can
liberate 1 mmol of ammonia
per min under the assay conditions.
2.6 Localization of the activity of urease immobilized on Porous Chitosan Membrane
by X-ray microanalysis
To estimate the uniformity and distribution of immobilized enzyme in microcosmic, X-ray
microanalysis was used to localize the activity of urease immobilized on the surface and
the inside of porous chitosan membrane.
2.6.1 Incubation of immobilized urease
To localize the activity of urease immobilized on porous chitosan membrane by X-ray
microanalysis, immobilized urease would be incubated. The incubation was achieved by the
method of Ma et al. [23]. Three pieces of 0.5 cm2 of immobilized
urease (sample A, B and C) were washed with redistilled water and Tris-HCl buffer (pH7.0)
by ultrasonic wave. Sample B was boiled in redistilled water for 1 h to inactivate as the
blank. Both of sample B and C were incubated together in the incubated solution containing
1 ml of 0.5 % BaCl2, 4 ml of Tris-HCl buffer (pH7.0), 2ml of 1% urea and 3 ml
of redistilled water at 37ºC for 20 min. Finally the sample B and C incubated were washed with
redistilled water thoroughly. Sample A did not be incubated in the incubated solution as
the blank. All the samples (A, B and C) were dried in a desiccator.
2.6.2 X-ray microanalysis of immobilized urease incubated (sample A, B and C)
To compare the difference of the activity of urease on Sample B and C, they were mounted
side-by-side on a sample stub with double-surface scotch tape and sample A was mounted on
another sample stub. To examine the distribution of immobilized urease on the inside of
porous chitosan membrane, the peeling sample was obtained from sample C by the peelback
method [24]. All the above samples was coated with sputtered carbon. Coated
samples were imaged and photographed by employing a scanning electron microscope (KYKY—1000B SEM) and the analytical conditions were: 25 kV accelerating
voltage, tilt angle 30°, take-out angle 35°, and working distance 20 mm. The X-ray
energy spectrums were collected by energy dispersive spectrometer (Thermo NORAN-Vantage
X-ray EDS). The mappings of Ba on the surface and the inside of chitosan membrane were
collected by using Vantage DSI System.
3 RESULT AND DISCUSSION
3.1 The structure and morphology of porous chitosan membranes
Porous chitosan matrix prepared by lyophilization has low mechanical strength [25],
and chitosan membranes using PEG as porogen could not have good macroporous structure [26].
To obtain porous membranes with desired pore structure and good strength, we prepared
porous membranes using dibutyl phthalate as porogen. The surface structures of 4 kinds of
membranes with different pore diameters ranging from 0.3-70mm were showed in Fig.1. It could be seen that the pores were
crowded uniformly on the surface of membranes. The cross-sections of 4 kinds of membranes
were showed in Fig. 2. As we see, while the sample 1 and the sample 4 contained smaller
thoroughfare pores, other two kinds of membranes contained crowded thoroughfare pores on
the cross-section. This indicates that the porous chitosan membranes possessing dense and
thoroughfare pores can be prepared by this method, and the pore diameter and structure of
membranes can be controlled by different conditions.
3.2 The distributing of pore diameter of porous chitosan membranes
The distributing histograms of pore diameter of the samples 1-4 were determined by image
analysis system of particle size. Their ranges of pore diameters were 5-70mm, 2.0-50mm, 1.5-9.5mm and 0.3-1.9mm respectively. Their mean pore diameter values were 15.542mm, 9.678mm, 3.566mm and 0.560mm respectively. It showed that the ranges of pore diameters were
concentrical and pore diameters were uniform.
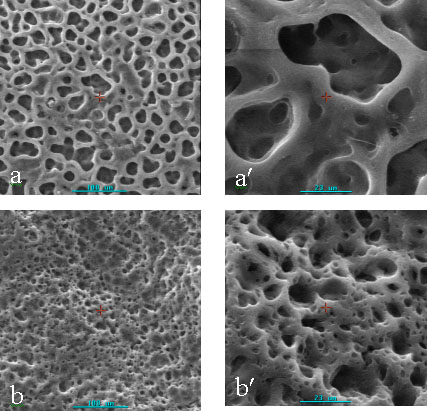
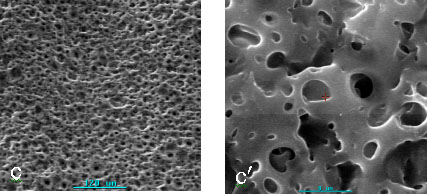
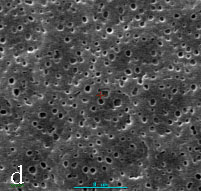
Fig.1 Scanning electron
micrographs of surface of porous chitosan membranes with different pore diameters (sample
1-4)
a,a' : sample1 (mean pore diameter: 15.542mm);
b,b' : sample2 (mean pore diameter: 9.678mm);
c,c' : sample3 (mean pore diameter: 3.566mm );
d: sample4 (mean pore diameter: 0.560mm).
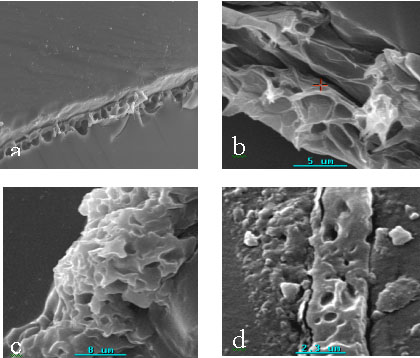
Fig.2 Scanning
electron micrographs of cross-section of porous chitosan membranes with different pore
diameter (sample 1-4)
3.3 The activity of urease
immobilized on porous chitosan membrane
In this investigation, urease was immobilized on porous chitosan membrane with 3.566mm of average pore diameter. It was
showed that the activity of urease immobilized on porous chitosan membrane was 17.22 U/cm2.min,
and 5 times as large as that of urease immobilized on non-porous chitosan membrane (3.6
U/cm2.min). This is mainly due to the larger surface area of porous chitosan
membrane than the latter.
3.4 Localization of the activity of urease immobilized on porous chitosan membrane
The amount of protein coupled onto the matrix and the activity of the immobilized enzyme
is dependent on the particular enzyme, the support and the coupling method involved [27].
The general determination of the enzyme activity shows only the amount of macroscopic
enzyme activity. To estimate the effects of immobilized conditions on the activity of
immobilized enzyme in microcosmic, X-ray microanalysis was used to localize the activity
of urease immobilized on porous chitosan membrane. BaCl2 and urea were selected
as capture and substrate respectively. Substrate was hydrolyzed by immobilized urease to
produce NH3 and CO2 in Tris-HCl buffer (pH7.0), and the latter was
captured by BaCl2 to form BaCO3 precipitate. The precipitation
deposited on the active site of immobilized urease. So Ba assigns the active site for
urease on carriers. Deposited Ba emitted characteristic X-ray by exciting of electron beam
and the X-ray energy spectrums were collected by X-ray energy dispersive
spectrometer(EDS). The reaction scheme is represented below.
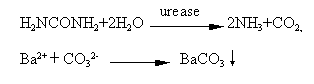
Elemental maps were generated for the samples. Fig.3 is the X-ray
energy spectrum of immobilized urease non-incubated (sample A) and P elemental was present
on it. This shows that P was absorbed or coupled on the immobilized urease and it is
difficult to be washed free. P was observed while Ba was absent on the X-ray energy
spectrum of immobilized urease destroyed by boiling incubated (sample B). Because the
activity of urease immobilized on sample B was destroyed by boiling and no urea was
hydrolyzed to produce BaCO3, the peak of Ba was absent. Concurrently, both P
and Ba were observed on sample C (immobilized urease incubated) and showed in Fig.4. This
indicates that the substrate urea was hydrolyzed by immobilized urease to produce CO2
that was captured by BaCl2 to form BaCO3 precipitate immediately.
SEM photographs and elemental mappings of Ba on the surface of the sample cohering of
sample B and C were showed in Fig.5. Ba distributed on the surface of membrane (sample C)
uniformly and it did not be observed on sample B. It is shown that the method localizing
of the activity of urease immobilized on porous chitosan membrane by X-ray microanalysis
is practicable and reliable.
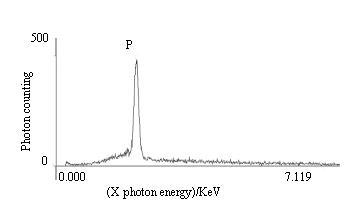
Fig.3 The X-ray energy spectrum of immobilized urease
without being incubated (sample A)
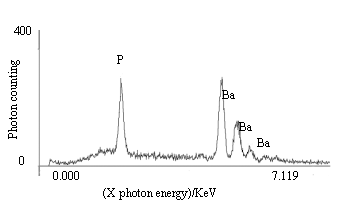
Fig.4 The X-ray energy spectrum of immobilized urease incubated (sample C)
3.5 The distribution of the activity
of urease on the porous chitosan membrane
SEM photograph and elemental mapping of Ba on the surface of immobilized urease
incubated (sample C) were showed in Fig. 6. Ba distributed on the surface of membrane
uniformly. SEM photograph and elemental mapping of Ba on the inside were collected from
the peeling sample obtained from sample C by the peelback method and showed in Fig.7. It
shows that Ba distributed not only on the surface of membrane but also on the inside
uniformly. These indicate that activated urease was immobilized uniformly on the surface
and inside of the porous chitosan membrane and the pores of chitosan membrane are
unimpeded.
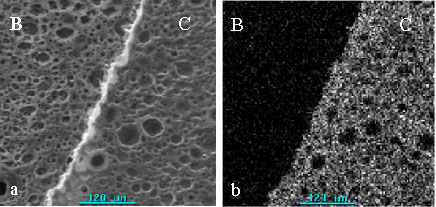
Fig.5 SEM photograph and mapping of Ba on the surface of
porous chitosan membranes incubated (sample B and C).
(a): SEM photograph. (b): Mapping of Ba on the surface of porous chitosan membranes.
Part B: immobilized urease destroyed by boiling (sample B), Part C: immobilized urease
without destroying by boiling (sample C).
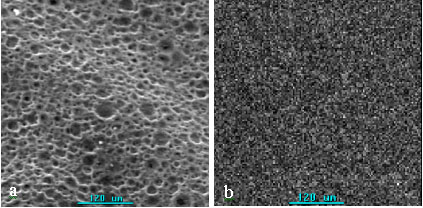
Fig.6 SEM photograph and mapping of Ba on the surface of porous chitosan
membrane incubated (sample C)
(a): SEM photograph; (b): mapping of Ba on the surface of porous chitosan membrane
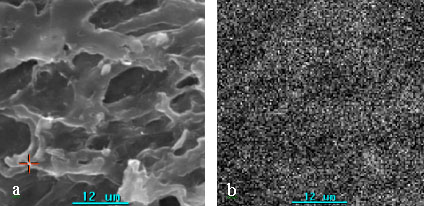
Fig.7 SEM photograph and mapping of Ba on the inside of immobilized
urease incubated (peeling sample of sample C)
(a): SEM photograph ; (b): mapping of Ba on peeling sample.
4 CONCLUSIONS
A novel method was developed to prepare porous chitosan membranes, using dibutyl
phthalate as porogen and the process is simple and easy. The porous chitosan membranes
possess controlled pore sizes, thoroughfare pores. X-ray microanalysis was used to study
the localization of the activity of urease immobilized on the porous chitosan membrane.
Investigation shows that porous chitosan membranes possess larger surface area and more
amount of urease immobilized than on non-porous chitosan membrane. The distribution of
activated enzyme was uniform on the surface and the inside of porous chitosan membrane.
REFERENCES
[1] Ma J B, Wang H J, He B L et al. Biomaterials, 2001, 22: 331-336.
[2] Li J L, Pan J L, Zhang L G et al. Biomaterials, 2003, 24: 2317-2322.
[3] Woong T, Chunga, Yang J et al. Biomaterials, 2002, 23: 2827-2834.
[4] Mi F L, Shyu S S, Wu Y B et al. Biomaterials, 2001, 22: 165-173.
[5] Mi F L, Wu Y B, Shyu S S et al. J. Membr. Sci., 2003, 212: 237-254.
[6] Kwunchit Oungbho, Bernd W. Müller. Int. J. Pharm., 1997, 156: 229-237.
[7] Lai H L, Abu’Khalil A, Duncan Q.M. Int. J.
Pharm., 2003, 251: 175-181.
[8] Leffler, C.C., Müller, B.W. Int. J. Pharm., 2000, 194: 229-237.
[9] Chow KS, Khor E. Biomacromolecules, 2000,1:61-67.
[10] Zeng X F, Ruckenstein E. Ind. Eng. Chem. Res., 1998, 37: 159.
[11] Zeng X F, Ruckenstein E. J. Membr. Sci., 1998, 148: 195-205.
[12] Zeng X F, Ruckenstein E. J. Membr. Sci., 1999, 156: 97-107.
[13] Steenkamp G C, Keizer K, H.W.J.P. et al. J. Membr. Sci., 2002, 197: 147-156.
[14] Zeng M F, Fang Z P, Xu C W. J. Membr. Sci., 2004, 230: 175-181.
[15] Júlia M. C. S. Talanta, 1998, 47: 183–191.
[16] Krajewska B. J. Chem. Tech. Biotechnol., 1990, 48: 337-350.
[17] Cetinus A. Enzyme and microbial technology, 2000, 26: 497-501.
[18] Amorim R. V. S. Bioresource Technology, 2003, 89: 35-39.
[19] Tan T W. J. of Molecular catalysis B. Enzymatic, 2002, 18: 325-331.
[20] Wang G.. Bioelectrochemistry, 2002, 57: 33-38.
[21] Roosevear A., Kennedy J.F., Cabral J.M.S. Immobilized enzymes and cells. Bristol,
Adam Hilger, 1987, 95.
[22] Weatherburn, M.W. Anal. Chem., 1967, 39: 971-974.
[23] Ma X L, Yao Z H. Spectroscopy and Spectral Analysis, 2005, 25 (3): 456-459.
[24] Linda C. Sawyer and David T. Grubb. Polymer microscopy. London; New York; Chapman and
Hall,1987, 85-86.
[25] Sundararajan V. Madihally, Howard W.T. Biomaterials, 1999, 20: 1133-1142.
[26] Ruckenstein E, Zeng X F. J. Membr. Sci., 1998, 142: 13-26.
[27] Zaborsky. O. Immobilized Enzymes. CRC Press, Cleveland, OH, 1973, 49-50.
多孔壳聚糖膜的制备表证及其固定化脲酶活性的 X射线微区分析
马晓莉1,2,姚子华,史大刚
(1河北大学化学与环境科学学院,河北省分析科学技术重点实验室 保定,071002;2河北省职工医学院,保定,071000)
摘要 以邻苯二甲酸二丁酯为致孔剂,制备了多孔壳聚糖膜,用作固定化脲酶的载体。利用SEM对多孔膜的表面和断面结构进行了表证,并利用SEM的粒度分析系统对多孔膜的孔径分布和平均孔径进行了分析。结果显示:多孔壳聚糖膜孔径范围为0.3mm - 70mm,平均孔径为0.560mm - 15.542mm。利用X射线微区分析方法,对多孔壳聚糖膜固定化脲酶的活性进行了定位分析:以BaCl2为捕捉剂,以尿素作为底物,在Tris-HCl缓冲溶液中,底物经固定化脲酶催化水解产生NH3和CO2,后者和捕捉剂反应生成BaCO3沉淀,
沉积在固定化脲酶的催化活性部位,借此确定其催化活性部位。结果表明:多孔壳聚糖膜通透性好,表面积大,载酶量高;多孔壳聚糖膜固定化脲酶的酶活为17.22
U/cm2.min ,是无孔膜固定化脲酶的5倍;脲酶不仅均匀分布在膜的外表面,膜内的孔眼中也有酶活,且分布均匀。
主题词 多孔壳聚糖膜,SEM,X射线微区分析,固定化脲酶
|