Structure and properties of latex
particles with different glass-transition temperature
Yang Huan, Zhang Jide, Zhu
Yangrong, Yang Mujie
(Department of Polymer Science and Engineering, Zhejiang University, Hangzhou 310027,
China)
Received Jun. 13, 2005.
Abstract Self-crosslinkable
core-shell latex was synthesized, and the influence of the hydrophilic core Tg
on the latex structure and the film properties was studied, then the film formation
mechanisms were suggested. Lower core Tg helps the migration of the core
polymer to the outer crosslink layer and increases the gel content and compatibility of
the film with two phases. The latex with higher core Tg have more obvious
core-shell structure.
Keywords latex particles, core-shell structure, self-crosslink, glass-transition
temperature, particle structure, IPN
1 INTRODUCTION
Recently, the coatings industry has been forced to develop environmentally friendly
coatings with less or ultimately zero VOCs (volatile organic compounds). Latex coatings
have been promised to reduce the VOCs emitted from the coatings. However, normal latex
coating, such as acrylic latexes, has relatively poor water resistance and unsatisfactory
film hardness etc., which limit their practical application in many fields. In order to
improve their properties, the applications of preparation technology of the core-shell
structured latex and self-crosslink technology in emulsion polymerization have been
attracting more and more interest in recent years. For the core-shell structure latex, the
optimum glass-transition temperature (Tg) of the latex particle could either
improve latex film hardness or favor formation of film [1-3]. For the
self-crosslink technology in emulsion polymerization, introducing the reactive groups onto
latex polymer, self-crosslinkable system could be obtained, which not only enhances the
physical and chemical integrity of latex films, but also makes the film formation process
complicated [4 -7]. Diacetone acrylamide (DAAM) has got a lot of attentions
recently, because that it can be copolymerized with acrylate easily and react with adipic
dihydrazide (ADH) by weak acidity or alkalescence as catalysts at room temperature to form
self-crosslinkable system [8], so DAAM and ADH were used in this study.
In this paper, self-crosslinkable core-shell latex was synthesized
through two-stage emulsion polymerization with acrylic monomers and DAAM. The influence of
the hydrophilic core Tg on the latex structure and the film properties was
investigated. The formation mechanisms of latex film so obtained were suggested.
2 EXPERIMENTAL
2.1 Materials
Styrene (St), n-butyl acrylate (BA), methyl methacrylate (MMA), ethylhexanoic
acid (EHA), and acrylic acid (AA) were obtained from Shanghai Wulian Co., China.
Hexanediol diacrylate (HDDA), DAAM, and ADH were purchased from Hangzhou Xinghua Fine
Chemical Co., China. Sodium dodecylsulfate (SDS), polyoxyethylene (10) octylphenyl ether
(OP-10) as composite emulsifuers, and APS as initiator were bought from Wenzhou Dongsheng
Chemical Co., China. Sodium hydrogencarbonate (NaHCO3) as buffer was got from
Shanghai Hongguang Chemical Plant, China. All the above materials were used without
further purification. Distilled and deionized water was used throughout the work.
2.2 Preparation of self-crosslinkable core-shell latex
Emulsion polymerization was performed at 75ºC in a conventional thermostated
three-necked glass reactor equipped with a mechanical stirrer and a reflux condenser using
a two-stage semi-continuous addition of stable emulsions of the core and shell monomers.
The first-stage copolymerization monomers include EHA、MMA and DAAM. According to Fox equation, we modified the ratio of
MMA to EHA to vary the Tg of the core polymer [9]. A constant amount of DAAM was introduced to make the latex
cross-linkable with ADH and hydrophilic. St, BA, AA and HDDA were copolymerized during the
second-stage and the quantity of each monomer was constant. The ratio of first-stage
monomer/second-stage monomer is 1/1(wt). The interval of the
two stage polymerization was 30min. Starve-feed condition was applied (~10g monomer per
1h) throughout the second-stage polymerization. When monomers addition was completed, the
temperature was increased to 80ºC and reacted for 30min, then 5g of initiator
solution (1 wt %) was fed. After 1 hour, another 5g of the initiator solution (1 wt %) was
added and polymerization was continued for a further 30min at 85ºC. The obtained
system was cooled to 40ºC to
adjust the pH value to ~7.5 by adding ammonia. The molar quantity of ADH added to latex
was half amount of that of DAAM.
2.3 Characterization of the latex and film
Particle morphology was investigated by transmission electron microscopy (TEM).The
emulsion was dispersed in the pure water, dropped on the holey copper net, and then
treated with a 2 wt % aqueous solution of phosphotungstic acid as a negative stain for TEM
(JEM 1200EX).
The particle size of the latex was determined by dynamic light
scattering (DLS) using a 90 Plus particle size analyzer (Brookhaven Instruments Corp.)
equipped with diode laser operating at 658.0 nm at 20°C after the latex was diluted by
deionized water.
The latex viscosity was measured by a NDJ-79 rotational viscometer
(Tongji Mechanical & Electronic Plant).
Gel content (%) of the latex film is calculated from Eq. (1).
Gel content (%) = (w1/
w0) × 100
(1)
Where w0 and w1 are the weights of
the latex film before and after swollen in acetone at room temperature for 24h and then
carefully dried. The latex films were formed with the aid of Texnaol at 40ºC for 36h in a poly (tetrafluoroethylene) mold after
blended with ADH, then at -0.1MPa for 24h, and the thickness is ~0.5mm. The films were
also used for turbidity experiments.
The turbidity t is defined as the light intensity reduction per unit penetration
length in the sample, L can be determined from the ratio of the transmitted light
intensity, It to the incident intensity, I0:
t = (-1/L) ln(It/I0)
(2)
The absorbance, It/I0
of the films was performed with a Cary 100 Bio Ultraviolet-visible light spectroscopy at
400nm. The conversion from absorbance to turbidity was performed by using Eq. (2).
3 RESULTS AND DISCUSSION
3.1 Effect of core Tg to particle morphology and viscosity of latex
The design of latex particle Tg is an important factor for obtaining the
core-shell structured latex particles. Figure 1 shows the TEM image of latex particle with
different core Tg. It is seen that the latex particles with higher core Tg
(30ºC) exhibit obvious core-shell structure in comparison to that with lower core Tg
(-30ºC). Moreover, its shell layer is more integrated and more uniform in the
thickness. The latex particles with lower core Tg (-30ºC) have not more integrated shell layer, which may
be due to the migration of the hydrophilic core polymer to the water-latex interface [10].
Figure 2 shows latex particles size and latex viscosity as the function
of the core Tg. No more change of the size of the latex particles was observed
with increasing the core Tg. The values of volume mean diameters are between
96nm~103nm, and the polydispersity values are below 0.042. However, at the same test
temperature (11ºC), the viscosity of the latexes with 44% solid content value
decreases obviously along with increase of the core Tg. For this case, when the
core Tg decreases, the polymer segment motion ability increases, and the
migration of the hydrophilic core polymer to the outer layer may become more and more
easily, at the same time, the concentration of hydrophilic secondary amine which is from
DAAM on the water-latex interface would rise too, thus increases the volume fraction that
latex particles occupy because of the hydration effect. According to Einstein's viscosity
law [11], the higher volume fraction that particles occupy increases the system
viscosity.
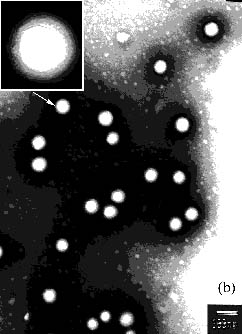
Figure 1 TEM micrographs of latex particle with different core Tg: (a) -30ºC,
(b) 30ºC
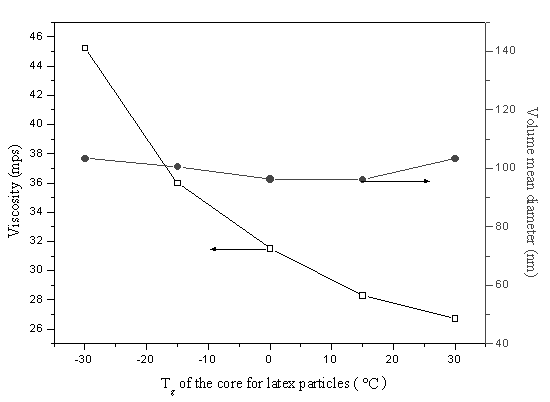
Figure 2 Influence of core Tg of the latex
particles on viscosity of latex (at 11ºC) and particle size
3.2 Effect of core Tg to the film
properties
Latex films formed from latex prepared blended with ADH have network structure,
because film formation process is accompanied with the crosslinking reaction between
ketone carbonyl and hydrazide. The quantity of DAAM introduced into emulsion
polymerization was consistent, so the invariable value of the gel content was expected.
But the practical value varies along with the core Tg. The results of the
swelling experiments are shown in Figure 3. When Tg of the core polymer is the
lowest (-30ºC), the gel content
of the film is the highest (81.4%), and with the increase of the Tg, the value
decreases to 76.5% at 15ºC. This decreasing trend should be related to the core
polymer's migration to the outer layer. This migration may result the formation of semi
interpenetrating network (semi-IPN) structured latex particle because of the crosslink of
the shell layer, and after addition of ADH, interpenetrating network (IPN) could be
obtained finally. The lower interpenetration degree between core and shell layer, the less
contribution the network of the original shell would be made to the final IPN, and lower
gel content value would be obtained. The gel content increases at 30ºC of the core Tg, which might be explained
by that the embedment of the ketone carbonyl in the inner latex helps to make the polymer
diffusion across the intercellular preceding cross-linking[12], so the further
reaction would not be hindered.
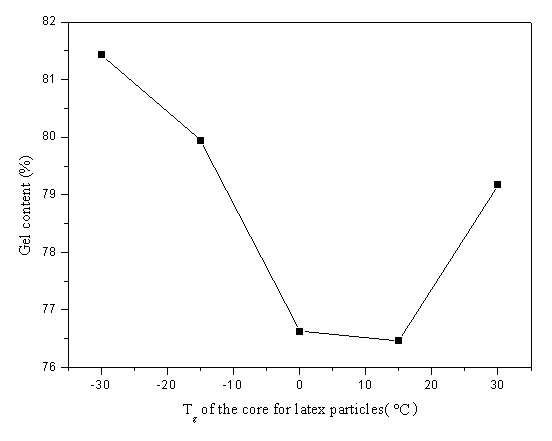
Figure 3 Gel content (%) of the film formed from latex
with different core Tg blended with ADH
Formation of the IPN could
reduce the contrast between different phases in material, and helps to have better
compatibility. The turbidity t has been used to characterize the compatibility of the two-phase
materials because of the phases with different refractive indexes [13]. Higher
turbidity shows lower compatibility. Along with the increase of the core Tg, t of the film presents the same trend
which increases from 1.428mm-1 at -30ºC to 3.709mm-1 at 30ºC (Figure 4). The lower turbidity value should be related to the
higher interpenetration degree between the core with the lower Tg and the outer
layer, and when core Tg becomes higher, more original shell network could not
play a part in the formation of the final IPN and might be separated in the network in
small phases, so higher turbidity value is obtained. For core-shell structured latex,
which is with two phases, more uniform film with IPN structure could be obtained by proper
design of the latex particle, which is meaningful for improvement of the performance of
film.
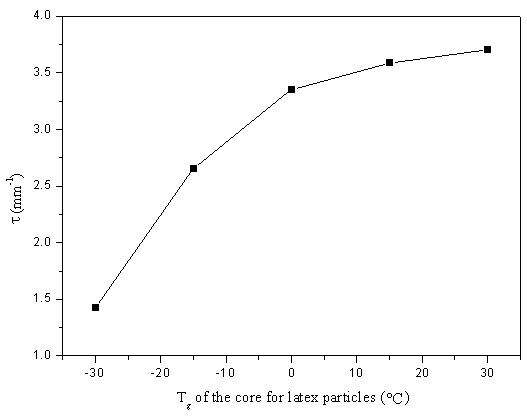
Figure 4 Turbidity of the film formed from latex with
different core Tg blended with ADH
3.3 Suggestion of film formation mechanism
According to the discussion on the structure of the latex and the film properties, two
film formation mechanisms were suggested, which is depicted schematically in Figure 5. For
lower Tg of the core, typically -30ºC, semi-IPN structured latex particle could be obtained because of the
migration of hydrophilic core polymer to the outer cross-linked layer. A part of ketone
carbonyl would be located at the water-latex interface. With the evaporation of water,
particles coalesce and film is formed, being accompanied with the crosslinking reaction
and IPN film was obtained finally. For higher Tg of the core, typically 30ºC, it may be difficult for the core polymer migration
to the water-latex interface, and the particle is core-shell structure. Cross-linkable
ketone carbonyl is embedded in the core. During the process of film formation, particles
come into contact and deform, and the original shell would fragment and separate in the
final network in small phases.
 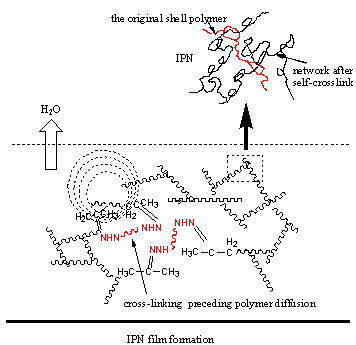
a
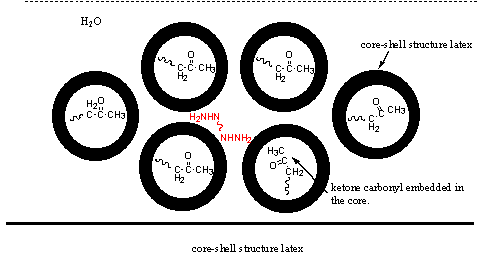 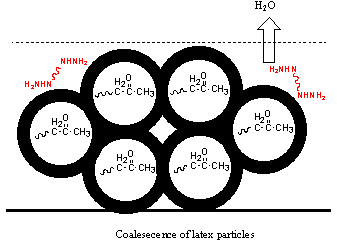
 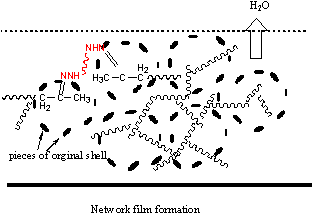
b
Figure 5 a: film formation process for
low core Tg latex; b: film formation process for high core Tg latex.
4 CONCLUSION
The migration degree of the hydrophilic core polymer to water phase was influenced by
the Tg of the core and it increased with the Tg decrease. Semi-IPN
structured latex could be obtained at the higher level of interpenetration of the core and
the network structured shell, and IPN film was obtained finally after film formation and
crosslinking reaction. When core Tg became higher, the level of the
interpenetration lowered, more obvious core-shell structured latex particles were
obtained.
REFERENCES
[1] Mazuel F, Bui C, Charleux B, et al. Macromolecules, 2004, 37: 6141
[2] Devon M, Gardon J, Robert G, et al. J. Polym. Sci., 1990, 39: 2119
[3] Eliseea V I. Progress. Org. Coating, 1985, 13: 195
[4] Winnik M A. J. Coat. Tech., 2002, 74: 49
[5] Geurink P J A, Van Dalen L, Van der Ven L G J, et al. Prog. Org. Coat., 1996, 27: 73
[6] Aradian A, Raphaël E, de Gennes P –G.
Macromolecules, 2000, 33: 9444
[7] Aradian A, Raphaël E, de Gennes P –G. Macromolecules, 2002, 35: 4036
[8] Coleman L E, Bork J F, Wyman D P, et al. J. Polym. Sci.: Part A, 1965, 3: 1601
[9] Fox T G, Flory P J. J. Appl. Phys., 1950, 21: 581
[10] Lee D I, Ishikawa T. J. Polym. Sci., Polym. Chem. Ed., 1983, 21: 147
[11] Seymour R B, Carrher C E. Polymer Chemistry. An introduction. Lagowski, J. J., Ed.
New York: Marcell Dekker, 1998
[12] Liu R, Winnik M A, Stefano F D, et al. Macromolecules, 2001, 34: 7306
[13] Sperling L H. Interpenetrating Polymer Networks and Related Materials. New York:
Plenum Press, 1981
具有不同玻璃化转变温度乳胶粒子的结构与性能
杨欢,张继德,朱杨荣,杨慕杰
(浙江大学高分子科学研究所,中国杭州310027)
摘要 本文合成了可自交联的具有核壳结构的乳胶粒子,研究了亲水性核的玻璃化转变温度对于乳胶粒子结构和膜性能的影响,并提出了成膜机理。较低的核的玻璃化转变温度有利于核高分子向交联的壳层的迁移,增加了具有两相结构的膜的凝胶含量,提高了其相容性。当核的玻璃化转变温度较高时,乳胶粒子具有更为明显的核壳结构。
关键词 乳胶粒子,核壳结构, 自交联, 玻璃化转变温度, IPN
|