Adsorption kinetics of basic
fuchsine and auramine lake yellow O on expanded graphite
Pang Xiuyan1,
Lv pu1, Gong fei1, Ren Haili1, Liang Xiuhua2
(1College of Chemistry and Environmental Science, Hebei University, Baoding,
071002; 2School of Engineering Technology, Hebei, Baoding, 071002)
Abstract In order to investigate the
adsorption kinetics of dyes Basic fuchsine (BF) and Auramine lake yellow O
(ALYO) on expanded graphite (EG), EG was prepared with 50 mesh crude graphite after
chemical oxidation intercalation of potassium permanganate and vitriol. Based on pseudo
first-order and second-order kinetic model, the adsorption models of EG for the two dyes
are discussed. Adsorption rate and activation energy of BF and ALYO on EG are calculated,
and the rate-limiting step for the adsorption process based on the second law of Fick are
investigated. Kinetic studies show that the kinetic data are well described by the pseudo
second-order kinetic model. Equilibrium adsorbance and initial adsorption rate increases with the increase of the initial dyes
concentration. Adsorption process of BF on EG holds small adsorbing activation energy. The
internal diffusion appears to be the rate-limiting step for the adsorption
process.
Keywords Expanded graphite, adsorption kinetics, rate-limiting
step, Basic fuchsine, Auramine lake yellow O
1. INTRODUCTION
As a main source of industrial wastewater, colored wastewaters require pretreatment
for color prior to disposal into
receiving water bodies or publicly owned treatment works. While both biological and
physical/chemical methods have been employed for dyes removal, the former method applied
has not been very successful, due to the essential non-biodegradable nature of most of the
dyes.Adsorption is a kind of effective measure, active carbon [1-4], anion
exchange resin [5], active sludge [6, 7], peat, steel plant slag and
fly ash [8] have been reported to be employed for the treatment of dyes.
According to Walker's adsorption experiment of acid dyes on active carbon [1],
micropore structure was found to be redundant in the adsorption of large molecular weight
compounds such as dyes, and only 14% of the total specific surface of the activated carbon
is available for adsorption due to the high molecular area and aggregation of the dye.
Expanded graphite (EG) is a kind of eco-material.
It attracts attentions of scientists and engineers as an absorbent with a high sorption
capacity for organic materials, such as heavy oil and biomedical molecules [9],
because of its large pores structure. But it has been reported not so much about its
application in adsorbing of dyes from wastewater. Wang pressed the worm-like particles
into low-density plate of 0.1g/cm3. In the treatment of dye waste-water from
woolen mill, a decreasing rate of chemical oxygen demanded of 40% was obtained [10].
Research group of Hebei normal university carried out a thermodynamics study of dyes on
EG. They reported that the sorption capacity of dyes was influenced by various factors,
not only the expanded volume of EG, primary concentration of dyes, but also the amount of
EG and pH [11]. Study on the sorption of medium yellow GG on EG proved that the
decolored rate arrived to 97% [12]. However, a systematic adsorption kinetics
studies need to be carried out to enrich the sorption theory of EG.
Basic fuchsine (BF) and Auramine lake yellow O (ALYO) are two kinds of
dyes with tritane structure and biphenyl methane structure, respectively. They can
dissolve in water and often hold deep chroma. Both of them are popularly used in the
pigmentation of cotton textile, silk, leather, paper, oil, fat, paint, and so on. In this
paper, the sorption kinetic characteristics of BF and ALYO on EG are studied.
2. EXPERIMENTAL
2.1 Preparation and characteristics of EG
Expandable graphite was prepared with 50 mesh crude graphite (C) as material, KMnO4
as oxidant (KMnO4 : C = 0.15:1, mass ratio) and vitriol as intercalation
compound (H2SO4: C = 5:1, mass ratio, the mass concentration of
vitriol was 50%). EG was prepared with expandable graphite expanded in KSW heating oven at
900 ºC. Structural parameters of EG were characterized by expanded volume, specific
surface area and total pore volume. These data were detected with AutoPore II 9220 Mercury
Porosimeter (Micromeritics Inc. USA) under varying pressures of 0.58 PSIA to 59814.12
PSIA. The porosity characteristics were showed as Table. 1.
Table. 1 Structural
parameter of expanded graphite
Expanded
volume
(ml / g) |
Specific
surface area
(m2/g) |
Total
pore volume
(cm3/g) |
330 |
1044.99 |
30.1145 |
2.2 Sorbates
Dyes of BF with tritane structure and ALYO with biphenyl methane structure were
selected as reference compounds. Molecular structures and molecular weight of these dyes
are shown in Table. 2.
Simulated dye wastewaters were prepared by dissolving the different
dyes in distilled deionized water at various concentrations. Absorbance values were
recorded at the wavelength for maximum absorbance (lmax) corresponding to each dye and its solution was
initially calibrated for concentration in terms of absorbance units.
Table. 2
Chemical structure and molecular weight of dyes used for experiment
Dyes |
Structure |
Molecular weight |
lmax(nm) |
BF |
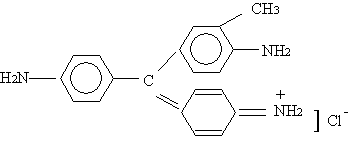
|
338.5 |
543 |
ALYO |

|
318.5 |
430
|
2.3 Adsorption of dye
In adsorption kinetics experiments, a series of desired dye concentration and of fixed
volume 100.0 ml were placed in vessels where they were brought into contact with EG at 5ºC,
25 ºC and 45ºC, respectively. The mass of adsorbent to volume of solution was
standardized at M/V=0.200 g/0.l l=2.00 g/l. The absorbency of dye solution
corresponding to different adsorption time was then analysed, and it was used to calculate
the solid phase dye concentration q. The amount of adsorbate captured by the adsorbant was
determined as the following:
q= V(C0-C)/M (1)
where:
q adsorption amount of adsorbate on adsorbent; mg/g
C0 initial concentration of dye in solution; mg/l
C concentration of dye in solution corresponding to a definite time;
mg/l
M mass of adsorbent; g
3 RESULTS AND DISCUSSION
3.1 Equilibrium time
The amount of dyes adsorbed is showed as a function of time in Fig.1~2. As showed in these
figures, adsorption occurs more rapidly at higher temperature: it takes only 3.0 h or 4.0
h to reach the adsorption equilibrium at 45ºC, while it takes about 24.0 h at 5ºC.
Initial dyes concentrations do not have a significant effect on the equilibrium time.
(a)
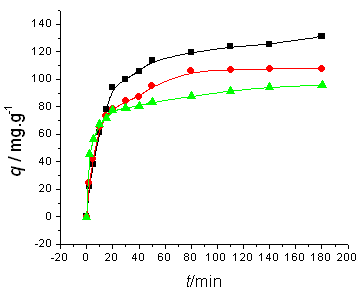
(b)
Fig. 1 Influence of initial BF
concentration and temperature on adsorption kinetics. Initial BF concentration is (a) 100 mg/l, (b) 200 mg/l; temperature(■) 5ºC, (●) 25ºC, (▲) 45ºC
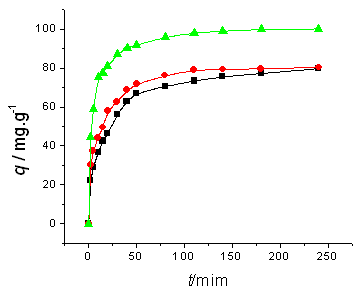
(a)
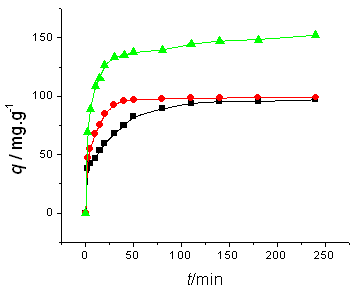
(b)
Fig. 2 Influence of initial ALYO concentration and temperature on
adsorption kinetics. Initial ALYO concentration is (a) 100 mg/l, (b)200 mg/l; temperature ( ■)5ºC, (●)25ºC, (▲)45ºC
3.2 Adsorption kinetic models
Both pseudo first- and second-order adsorption models were used to describe the adsorption
kinetics data [13, 14]. In both models, all the steps of adsorption
such as external diffusion, internal diffusion, and adsorption are lumped together, the
overall adsorption rate is proportional to either the driving force (as in the pseudo
first-order equation) or the square of the driving force (as in the pseudo second-order
equation).
First-order model:ln(qe-q)=lnqek1t
(2)
Second-order model:t/q=1/(k2 qe2)+t/qe
(3)
where:
k-adsorption rate constant (min-1 for first-order
adsorption, g·mg-1·min-1 for second-order adsorption)
t-adsorption time (min)
Since q reaches a plateau (qe) at equilibrium,
q values smaller than the 0.9qe were used for analysis. The plots
of ln(qe-q) versus t and t/q versus t were
used to test the first- and second-order models, and the fitting results are given in
Table. 3 and Table. 4. According to the correlation coefficients, second-order model gives
satisfactory fits. At the same time, the qe,cal obtained from the
intercepts is obviously inconsistent with the experimental data qe,exp
in the first-order model. In the second-order model, the qe,cal agrees
reasonably well with the experimental data. Thus, the second-order model is more suitable
to describe the adsorption kinetics data. Similar results were observed in biosorption of
dye Remazol Black B, RB2, PY2 on biomass and BBF on xerogel [15-17], anionic
dye adsorption on cross-linked chitosan beads [14, 18], and polyethylene glycol
(PEG) adsorption on zeolite [19].
Based on the second-order model, the initial adsorption rate and
half-adsorption time are estimated in Table. 5 and Table. 6 according to the following
equations:
u=kqe2 (4)
t1/2=1/(kqe) (5)
where:
u initial adsorption rate mg/(g·min)
t1/2 half-adsorption time min
Table. 3 Comparison of the
experiments and the kinetic models of BF adsorption on EG
C0 mg/l |
T
ºC |
qe,exp
mg/g |
First-order |
Second-order |
qe,cal
mg/g |
k1
min-1 |
r |
qe,cal
mg/g |
k2
g/(g·min) |
r |
100 |
5 |
104.36 |
47.17±1.20 |
0.0096±0.001 |
0.903 |
105.60±0.254 |
9.47±0.023 |
1.000 |
25 |
85.37 |
47.31±1.20 |
0.0210±0.002 |
0.938 |
88.50±0.872 |
11.3±0.111 |
1.000 |
45 |
73.65 |
32.56±1.15 |
0.020±0.0019 |
0.956 |
74.35±0.788 |
13.43±0.14 |
0.999 |
200 |
5 |
137.66 |
62.55±1.18 |
0.0098±0.001 |
0.922 |
139.28±0.550 |
7.18±0.028 |
1.000 |
25 |
110.36 |
58.25±1.19 |
0.022±0.0023 |
0.942 |
113.77±1.07 |
8.79±0.083 |
1.000 |
45 |
98.36 |
42.30±1.13 |
0.0168±0.002 |
0.952 |
97.37±1.18 |
10.27±0.13 |
0.999 |
The initial adsorption
rate is found to increase with the increase in the initial dyes concentration, but the
temperature has different influences on initial adsorption rate. To BF, it decreases with
the increase in temperature, and the value of ALYO increases with the increasing in
temperature. The influence of temperature and concentration on t1/2 is not
obvious.
The second-order rate constants listed in Tab. 3 and Tab. 4 are used to
estimate the activation energy of BF and ALYO adsorption on EG using Arrhenius equation:
Lnk=LnA-Ea/(RT) (6)
where:
A pre-exponential factor (g·mg-1·min-1)
Ea activation energy of adsorption (kJ/mol)
The slope of plot of ln k
versus 1/T is used to evaluate Ea, which was found to be 6.48–6.62 kJ/mol for BF adsorption on EG (listed in Table.5), and the
value of Ea for ALYO adsorption on EG was ignored for the reason of veracity (with the
correlation coefficients less than 0.9000). These values are smaller than that in the
literature where the activation energy was found to be 16.3-43.8 kJ / mol for the
adsorption of BBF on xerogel [16]. The results might be caused by the different
porous characteristics of EG and xerogel, and it could also be testified from the
different adsorption equilibrium time of 4.0 h on EG and 144.0 h on xerogel.
Table. 4 Comparison of the
experiments and the kinetic models of ALYO adsorption on EG
C0
mg/l |
T
ºC |
qe,exp
mg/g |
First-order |
Second-order |
qe,cal
mg/g |
k1
min-1 |
r |
qe,cal
mg/g |
k2
g/(g·min) |
r |
100 |
5 |
81.32 |
47.1±1.11 |
0.0147±0.001 |
0.970 |
82.6±0.91 |
12.1±0.132 |
0.999 |
25 |
82.36 |
36.5±1.19 |
0.0152±0.002 |
0.925 |
83.1±0.59 |
12.04±0.09 |
1.000 |
45 |
101.23 |
33.5±1.21 |
0.0171±0.002 |
0.931 |
101.8±0.3 |
9.82±0.033 |
1.000 |
200 |
5 |
98.36 |
53.4±1.15 |
0.0177±0.001 |
0.963 |
101.4±1.3 |
9.86±0.128 |
0.999 |
25 |
101.59 |
26.1±1.33 |
0.0136±0.003 |
0.800 |
100.7±0.4 |
9.93±0.042 |
1.000 |
45 |
154.36 |
53.8±1.18 |
0.0142±0.002 |
0.924 |
153.1±1.1 |
6.53±0.045 |
1.000 |
Table. 5 Kinetic parameters
for the second-order adsorption model of BF adsorption on EG
C0
mg/l |
T
ºC |
u
mg/(g·min) |
t1/2
min |
Ea
kJ/mol |
r |
100 |
5 |
103.6±0.248 |
1.01±0.002 |
6.48±0.044 |
1.000 |
25 |
82.35±0.811 |
1.04±0.01 |
45 |
72.96±0.77 |
0.95±0.04 |
200 |
5 |
136.06±0.537 |
1.01±0.004 |
6.62±0.229 |
0.998 |
25 |
107.05±1.00 |
1.03±0.009 |
45 |
99.35±1.21 |
0.990±0.012 |
Table. 6 Kinetic parameters
for the second-order adsorption model of ALYO adsorption on EG
C0
mg/l |
T
ºC |
u
mg/(g·min) |
t1/2
min |
Ea
kJ/mol |
r |
100 |
5 |
80.02±0.876 |
1.02±0.011 |
- |
- |
25 |
81.67±0.581 |
1.01±0.007 |
45 |
100.63±0.335 |
1.006±0.003 |
200 |
5 |
95.39±1.24 |
1.03±0.012 |
- |
- |
25 |
102.48±0.433 |
0.991±0.004 |
45 |
155.59±1.08 |
0.992±0.007 |
3.3 Internal diffusion analysis
The adsorption process on a porous adsorbent generally involves three stages: (i) external
diffusion; (ii) internal diffusion (or intra-particle diffusion); (iii) actual adsorption [19].
Quantitative treatment of experimental data may reveal the predominant role of a
particular step among the three that actually governs the adsorption rate. The adsorption
step is usually very fast for the adsorption of organic compounds on porous adsorbents
compared to the external or internal diffusion step [20], and it is known that
the adsorption equilibrium is reached within several minutes in the absence of internal
diffusion [21]. Thus, the long adsorption equilibrium time in experiments (3.0–24.0 h) suggests that the internal diffusion may dominate the
overall adsorption kinetics.
To provide definite information on the rate-limiting step, an internal
diffusion model based on Fick's second law is used to test if the internal diffusion step
is the rate-limiting step [22]:
q =kidt1/2 (7)
where:
kid internal diffusion constant, mg/(g·min1/2)
According to the internal diffusion model, a plot of q versus t1/2
should give a straight line with a slope kid and an intercept of zero if
the adsorption is limited by the internal diffusion process [22]. The
relationships between q and t1/2 at different temperature are
shown in Fig. 3 and Fig. 4. Initially in all the cases studied, a linear relationship
between q versus t1/2 with a zero intercept is found, suggesting
that the internal diffusion step dominates the adsorption process before the equilibrium
is reached.
(a)
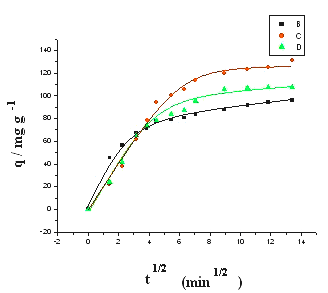
(b)
Fig. 3 Plot of q vs. t1/2
in internal diffusion model. Initial BF concentration is (a) 100 mg/l, (b) 200 mg/l; temperature (■) 5ºC,(●) 25ºC,(▲) 45ºC
(a)
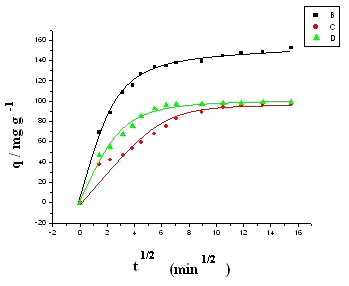
(b)
Fig. 4 Plot of q vs. t1/2
in internal diffusion model. Initial ALYO concentration is (a) 100 mg/l, (b) 200 mg/l; temperature (■) 5ºC,(●) 25ºC,(▲) 45ºC
To see this more clearly, two dimensionless
variables are defined, x and q:
x=q/qe;
q=t/t1/2 (8)
equation (8) can be rewritten as the following:
x=k'q1/2 (9)
where:
k' dimensionless internal diffusion
rate constant
x dimensionless adsorption amount
q dimensionless time
Fig. 5 and Fig. 6 show the plot of x versus q1/2, which
clearly show that all the data follow the same kinetics. This confirms that the adsorption
process can be described by a simple internal diffusion model. For the adsorption of
reactive dyes by activated carbon [23], the adsorption of methylene blue by
perlite [24], and the adsorption of BBF by xerogel [16], internal
diffusion were found to be the rate limiting step.
Fig. 5 Plot of x vs. q1/2 in internal diffusion model. Concentration refers to the initial
BF concentration
Fig. 6 Plot of x vs. q1/2 in
internal diffusion model. Concentration refers to the initial ALYO concentration
4 CONCLUSION
The use of Expanded graphite for the adsorption of organic dyes from aqueous solution
have been examined. The results are summarized as follows:
(1) The adsorption kinetics can be well described by the pseudo second-order kinetic
model. Initial adsorption rate increases with the increase in initial BF and ALYO
concentration. Temperature and initial dyes concentration do not have obvious influence on
t1/2.
(2) The equilibrium adsorption amount is found to increase with the increase in initial BF
and ALYO concentration. The internal diffusion of BF or ALYO into the EG is the
rate-limiting step of the overall adsorption process.
(3) Adsorption of BF on EG holds small adsorbing activation energy; the result might be
caused by the macroporous characteristics of EG.
Acknowledgements This study was
supported by Doctor Foundation of Hebei province Education Office (China, No.B2004402) and
Doctor Foundation of Hebei University. The authors gratefully acknowledge their support
during the study.
REFERENCES
[1]Walker G M, Weatherley L R. Chem. Eng. J, 2001, 83(3): 201.
[2]Namasivayam C, Kavitha D. Dyes and Pigments, 2002, 54(1): 47.
[3]Duggan O, Stephen J A. Water Sci. Tech, 1997, 35(7): 21.
[4]Attia A A, Rashwan W E, Khedr S A. Dyes and Pigments, 2006, 69(3): 128.
[5]Silke K, Anja K U, Martin J. Water Res, 2002, 36: 4717.
[6]Chu H C, Chen K M. Proc. Biochem, 2002, 37(6): 595.
[7]Chu H C, Chen K M. Proc. Biochem, 2002, 37(10): 1129.
[8]Jain A K, Gupta V K, Bhatnagar A S. J. Hazard. Mater, B, 2003, 101: 31.
[9] Kang F Y, Zeng Y P, Zhao H, et al. New Carbon Materials (Xinxing Tan Cailiao),
2003, 18 (3): 161.
[10]Wang L N, Chen X, Zheng Y P, et al. Guide J of Chinese Nonmeteal Mine Ind.
(Zhongguo Feijinshu Kuangye Daokan), 2004. 5: 59.
[11]Yang L N,. Hebei Normal University, MS thesis. 2004.
[12]Li J H,Yang L N, W G. Environmental protection
of chemical industry (Huagong Huanbao), 2004, 24 (S1): 374.
[13]Chiou M S, Li H Y. Chemosphere, 2003, 50(8): 1095.
[14]Chiou M S, Li H Y. J. Hazard. Mater, 2002, 93(3): 233.
[15]Aksu Z, Tezer S. Process Biochem, 2000, 36(5): 431.
[16] Wu Z J, Joo H, Lee K. Engineering Journal, 2005, 112(1-3): 227.
[17]Aksu Z. Biochem. Eng. J, 2001, 7(1): 79.
[18]Chiou M S, Ho P Y, Li H Y. Dyes and Pigments, 2004, 60(1): 69–84.
[19]Chang C Y, Tsai W T, Ing C H, et al. J. Colloid Interface Sci, 2003, 260(2):
273.
[20]Sarkar M, Acharya P K, Bhattacharya B. J. Colloid Interface Sci. 2003, 266 (1): 28.
[21]Mak S Y, Chen D H. Dyes and Pigments, 2004, 61(1): 93–98.
[22]Bhattacharyya K G, Sharma A. J. Environ. Manage, 2004, 71(3): 217–229.
[23]Yang X Y, Al- Duri B. J. Colloid. Interface Sci, 2005, 287(1): 25–34.
[24]Dogan M, Alkan M, Turkyilmaz A, et al. J. Hazardous Mater, 2004, 109(1–3):141.
碱性品红与碱性嫩黄O在膨胀石墨上的吸附动力学研究
庞秀言 吕溥 巩菲 任海丽 梁秀华#
(河北大学化学与环境科学学院 保定 071002;#河北省科技工程学校
保定 071002)
摘要 研究了碱性品红与碱性嫩黄O在以50目天然石墨为原料,高锰酸钾为氧化剂,硫酸为插层剂制备的膨胀石墨上的吸附特性。基于准一级、准二级动力学模型研究了两种染料在膨胀石墨上的吸附模型,计算了其吸附速率和吸附活化能;基于Fick第二定律,确定了吸附过程速率控制步骤。研究表明:两种染料在膨胀石墨上的吸附动力学模型为二级;平衡吸附量随染料初始浓度增加而增大;初始吸附速率随初始浓度的升高而增大;碱性品红在膨胀石墨上的吸附活化能较小;吸附过程为内扩散控制。
关键词
膨胀石墨,吸附动力学,速率控制步骤,碱性品红,碱性嫩黄O
|