Determination of resorcinol on glassy carbon
electrode modified by carbon nanotube and its application in wastewater analysis
Liang Shuxuan 1 , Yuan Chenguang1,
Zhang Huankun2, Yang Liu1,Sun Hanwen 1
(1College of Chemistry and Environmental Science, Hebei University, Baoding
071002; 2 Environmental
Science Academe of Heibei, Shijiazhuang, China)
Abstract Adsorptive
stripping method for the determination of resorcinol on multi-walled carbon nanotube
modified glassy carbon electrode (MWNT-GCE) was developed. Cyclic voltammetry was used in
the study of the electrochemical behavior of resorcinol on MWNT-GCE. The sharp peak of
resorcinol appeared at 0.6 V (vs. Ag/AgCl) with the differential pulse voltammetry (DPV).
The experimental parameters were optimized. Anodic peak currents were linear over the
resorcinol concentrations in the range of 5.5กม10-5-3.3กม10-3 g L-1
with a detection limit of 1.1กม10-5 g L-1 and relative standard
deviations of 3.30-6.94% for 7 determinations. The interference of organic and inorganic
species on the voltammetric response was also tested. The proposed method was applied to
the determination of resorcinol in tap water and simulative wastewater samples with an
average recovery of 95.2-104.8%.
Keywords Determination; Carbon nanotube; Modified glassy carbon electrode;
Differential pulse voltammetry; Resorcinol
1. INTRODUCTION
Resorcinol is an important industrial chemical
material used widely in the fields of rubber, plastics, and organic synthesis industries,
wood adhesives, fire retardants, and UV stabilizer, etc. It is derived from various resins
and tannins but most commonly by fusing sodium hydroxide with meta-benzene-disulfuric
acid. Global output of resorcinol in 2004 was about 41.83 million tons. This compound is a
moderate toxic substance and easily soluble in water[1]. There is a growing
need for developing highly sensitive, simple methods to detect resorcinol in the
wastewater at a low level. Various technologies for the detection of resorcinol have been
developed, such as high performance liquid chromatography (HPLC)[2,3],
thin-layer chromatography[4], spectrophotometry[5,6],
surface plasmon resonance[7], and biochemical methods, including enzyme[8],
bioluminescence[9], biosensor[10]. However, sample pre-treatment
processes involving separation, extraction, and adsorption are generally necessary, and
these steps are time-consuming and complex.
The chemical modification of electrode (CME) is a field of growing
interest in analytical chemistry. The modification of the electrode surface could promote
reactivity and selectivity to the base sensor, leading to the development of electrodes
for some specific applications[11,12]. It has been demonstrated that CME
possesses distinct advantages over conventional electrode in many application areas
including electrocatalysis and electrochemical sensors. One of the most important
properties of the CME, which has been the subject of considerable study, is their ability
to catalyze the oxidation or reduction of some compounds[13,14]. Carbon nanotube is of fullerene-related structures that consist of
graphite cylinder closed at either end with cap containing pentagonal ring. It is
promising as immobilization substance because of its significant mechanical strength, high
surface area, excellent electrical conductivity and good chemical stability. The main
advantages of a nanoparticle-modified electrode compared to a macroelectrode are high
effective surface area, mass transport, catalysis and control over local microenvironment[15].
In essence the small dimension of nanoparticle brings about convergent rather than linear
diffusion, resulting in a higher rate of mass transport to the electrode surface. However,
this can modify the appearance of the voltammetric signal due to the increased mass
transport overpotential. In view of the excellent properties of carbon nanotube, it has
been used to modify different electrodes for determination of chemical and biological
species[16].
Not many
electrochemical studies of resorcinol have been reported. Nasr et al.[17] and
Robson T. S. Oliveira et al.[18] reported the electrochemical oxidation of
resorcinol on boron-doped diamond anodes. Cyclic voltammetric study showed that the
current density of resorcinol was smaller and no reverse peak was observed. Wang group[19]
reported the method of simultaneous determination of hydroquinone, resorcinol, and
catechol by voltammetry with single-walled carbon nanotube (SWNT) modified glassy carbon
electrode. The detection limit of resorcinol was 3.3กม10-5 g L-1.
Each of these studies has reported the electrochemical behavior or the determination of
dihydroxybenzene isomers, but so far there is no report on the analysis of resorcinol by
voltammetry with multi-walled carbon nanotube (MWNT) modified glassy carbon electrode.
This work investigated a new method for the determination of resorcinol
by differential pulse voltammetry (DPV) using a glassy carbon electrode modified by
multi-walled carbon nanotube, which promoted better electron transfer between the
electrode surface and electroactive species in solution. The modified electrode exhibited
a remarkable accumulation effect for resorcinol and high sensitivity. The proposed method
also displayed good resistance to the interference from some organic and inorganic
compounds.
2. EXPERIMENTAL
2.1. Apparatus
The voltammograms were obtained by using a MEC-12B microcomputer-controlled multimode
electrochemical analyzer with an ATA-B rotating disk electrode and a three-electrode
set-up (Jiangfen Electroanalytical Instrumental Ltd., Jiangyan, China). The experiments
were carried in a three-electrode cell containing a glassy carbon electrode (GCE, 3 mm in
diameter) as working electrode, an Ag/AgCl electrode with saturated KCl as reference
electrode, and a platinum wire as counter electrode. The software package of
electrochemical analyzer system worked through a personal computer with data acquisition
routine. Repetitions of voltammetric measurements for each resorcinol concentration were
automatically controlled with a computer program in order to achieve good statistics of
the measurements. A KQ-50E ultrasonic cleaner (Kunshan Ultrasonic Instrument Company Ltd.,
Shanghai, China) was employed during the electrode preparation process. SiC sandpaper was
used to polish GCE.
Capillary electrophoresis (CE) determination was performed by an
Agilent 3D CE system with air-cooling and a diode array detector (Agilent Technologies,
Waldbronn, Germany) to validate the proposed electrochemical method. A 48.5 cm (40.0 cm to
the detector) กม 50 mm i.d.
uncoated fused-silica capillary (Agilent) was utilized. During the determination process,
capillary temperature and applied voltage were set at 20 กๆ, 15 kV respectively. UV detection was carried at 280 nm.
2.2. Reagents and solutions
An aqueous stock standard solution containing 1.1 g L-1 resorcinol was
prepared by dissolving 0.11 g resorcinol in 100 mL water. Suspension of 1.0 g L-1
multi-walled carbon nanotube was prepared by adding 50 mg MWNT(30~50 nm) into 50 mL water
and sonicated for 20 min. 0.2 mol L-1 sodium hydroxide (NaOH) was prepared to
activate electrode. pH=7.0 buffer solution of KCl, Na2HPO4, KH2PO4
was prepared by mixing 50 mL 0.2 mol L-1 KCl solution, 12.5 mL 0.1 mol L-1
Na2HPO4 solution, and 25 mL 0.2 mol L-1 KH2PO4
solution, then diluted to 100 mL. The buffer solution was used throughout this study,
unless stated otherwise.
All chemicals were of analytical-reagent grade. All solutions were
prepared using 18.3 MW water obtained from a Mili-Q purification system.
2.3. Preparation of the modified electrode
The GCE was previously polished with a piece of SiC
sandpaper, then thoroughly washed with doubly distilled water and anhydrous ethanol in the
ultrasonic cleaner for 5 min, respectively, and dried in air. MWNT films were prepared by
casting 50 mL aqueous dispersion of 1.0 g L-1 MWNT onto GCE, then dried under an
infrared light. The modified electrode was activated by immersing in 0.2 mol L-1
NaOH and then cleaned electrochemically by cycling potential scans for five cycles between
-1.5 to 1.5 V at a scan rate of 200 mV s-1 and accumulation potential of -1.5
V.
2.4. Analytical procedures
5 mL buffer solution (pH=7.0) was transferred
into a dry sample cell and resorcinol standard stock solution was added with definite
volume by a micropipette. The above solution was diluted to 10 mL with buffer solution and
deoxidized by bubbling with extra pure nitrogen for about 15 min before electrochemical
measurements. After a 15 s rest, the DPV method was applied from 0 V to 1.3 V to the
modified GCE (versus Ag/AgCl reference electrode). The pulse amplitude, pulse width,
accumulation time, accumulation potential was 50 mV, 50 ms, 60 s, -0.4 V respectively. The
measured data were recorded by a workstation with 10 mV intervals.
Cyclic voltammetry (CV) method was applied for acquiring qualitative
information about electrochemical reactions, the cyclic voltammograms were recorded by
applying a scan over the potential range from 0 V to 1.3 V to the GCE (versus Ag/AgCl
reference electrode). The accumulation time, accumulation potential, and scan rate were 60
s, -0.4 V, and 200 mV s-1, respectively. Data acquisition and treatment were
performed with a workstation.
3. RESULT AND DISCUSSION
3.1. Electrochemical response of resorcinol on WMNT modified glassy carbon electrode
The cyclic voltammograms of this system are
shown in Fig. 1. Anodic peak increased obviously after addition of resorcinol and this
result suggested that anodic peak of resorcinol was produced on GCE. The anodic peak
potential was at 0.6 V on the GCE in pH=7.0 buffer solution. Only one anodic peak was
observed and this result confirmed the irreversibility of the electrochemical reaction
under investigated conditions.
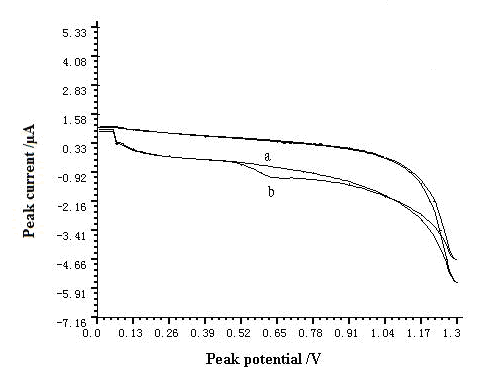
Fig. 1 Cyclic voltammograms of resorcinol in pH=7.0
buffer solution.
a-blank solution, b-5.5กม10-3 g L-1 resorcinol. The accumulation
time, accumulation potential, and scan rate were 60 s, -0.4 V, and 200 mV s-1,
respectively.
3.2. Influence of modified electrode
In our study, bare GCE, MWNT modified
GCE, and sodium montmorillonite modified GCE were
compared during the determination of resorcinol, respectively. The results were shown in
Fig. 2. According to the sensitivity of each electrode, MWNT modified GCE obtained a best
result.
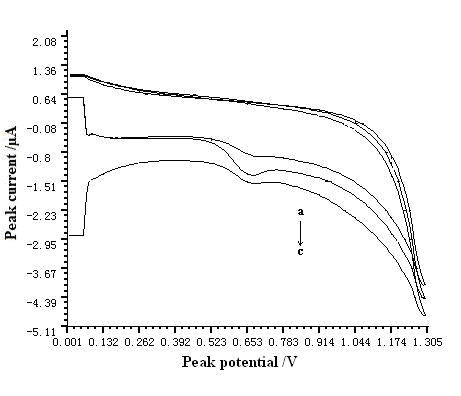
Fig. 2 Comparison of different
kinds of electrode. a - sodium montmorillonite modified GCE; b -
MWNT modified GCE; c- bare electrode.
(Conditions: pH of buffer solution was 7.0. The
accumulation time, accumulation potential, scan rate and the concentration of resorcinol
were 60 s, -0.4 V, 200 mV s-1, and 5.5กม10-3 g L-1,
respectively.)
3.3. The choice of buffer solution and pH
It was important for the chemically modified electrode to choose the buffer solution
and the pH of the buffer solution. A series of buffer solutions were tested. In 0.2 mol L-1
Na2CO3 + NaHCO3 (pH=9.5~11.5), NaOH + sodium citrate
(pH=4.0~6.0), and 0.2 mol L-1 NaOH solution, no peak appeared. In KH2PO4+NaOH
(pH=5.8~8.0), KH2PO4 + Na2HPO4 + KCl
(pH=6.6~7.8), a cathodic peak appeared. The peak height and shape in KH2PO4
+ Na2HPO4 + KCl were optimum, so we chose KH2PO4
+ Na2HPO4 + KCl buffer solution for subsequent studies.
Effect of pH on peak current and peak potential of resorcinol was
investigated in KH2PO4 + Na2HPO4 + KCl buffer
solution in the pH range from 6.60 to 7.80. The results were shown in Fig. 3. The
voltammetric peak potential for resorcinol became more negative with the increase of pH in
a roughly linear manner. This suggested that the concentration of hydrogen ions affected
the rate of reaction. The hydroxyl group dissociated gradually and shifted the peak to
more negative values as pH increased. The H+ concentration affected the peak
current of resorcinol obviously also. The peak current gave a maximum peak in pH=7.0
buffer solution. Thus, the buffer solution of pH=7.0 was selected as a suitable analytical
medium in this study. At pH=7.0, concentrations of KH2PO4, Na2HPO4,
and KCl were 0.05 mol L-1, 0.0125 mol L-1 and 0.1 mol L-1,
respectively.
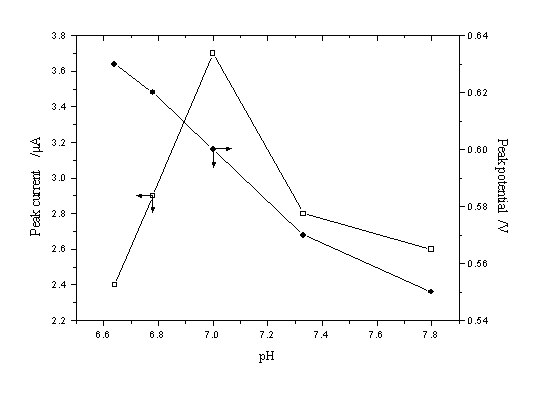
Fig. 3 Influence of pH of the KH2PO4 +
Na2HPO4+ KCl buffer on peak current and peak potential.
The accumulation time, accumulation potential, and scan rate were 60 s, -0.4 V, and 200 mV
s-1, respectively (5.5กม10-3 g L-1 resorcinol)
3.4. Influence of accumulation time and
potential
It should be mentioned that it was important to choose accumulation time and potential
when adsorption studies were undertaken, since both parameters could influence the degree
of adsorption of the resorcinol reaction. Bearing this in mind, the effect of accumulation
time and potential on anodic peak current was investigated by CV.
The peak current increased with the accumulation time in the range from
ca. 0 to 60 s. However, at about 60 s, further increase in the accumulation time couldn't
increase the amount of resorcinol at the electrode surface due to surface saturation, and
the peak current remained constant.
The effect of accumulation potential was examined by using 5.5กม10-3
g L-1 resorcinol at a constant time of accumulation of 60 s over the range from
0 to 0.7 V in pH 7.0 buffer solution. The results were shown in Fig. 4. The responses were
obviously influenced by the accumulation potential. The optimal accumulation potential was
0.4 V, which was chosen for further studies.
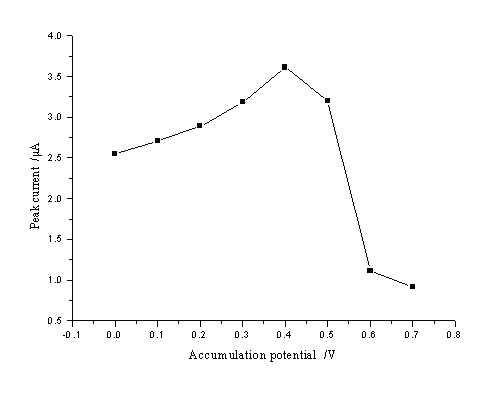
Fig. 4 Effect of accumulation potential on peak
current.
The accumulation time, accumulation potential, and scan rate were 60 s, -0.4 V, and 200 mV
s-1, respectively (5.5กม10-3 g L-1 resorcinol)
3.5. Reproducibility
The 5.5กม10-5~3.3กม10-3 g L-1 resorcinol was
determined repeatedly with the same electrode for 7 times. The average R.S.D. was
3.99~8.94%. It indicated that the reproducibility of the MWNT modified GCE was remarkable.
Voltammetric response of resorcinol (5.5กม10-5--3.3กม10-3 g L-1)
on MWNT modified GCE was plotted in Fig. 5.
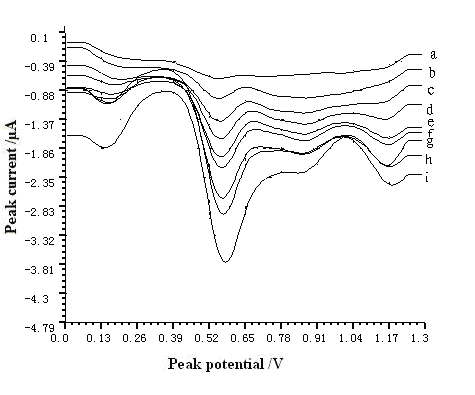
Fig. 5 Voltammetric response of resorcinol on MWNT
modified GCE. The pH, The accumulation time, accumulation potential, and scan rate were
7.0, 60 s, -0.4 V, and 200 mV s-1, respectively. (a) 5.5กม10-5 g L-1
(b) 2.2กม10-4 g L-1 (c) 4.4กม10-4 g L-1 (d)
6.6กม10-4 g L-1 (e) 8.8กม10-4 g L-1 (f)1.1กม10-3
g L-1 (g) 1.65กม10-3 g L-1 (h) 2.2กม10-3 g L-1
(i) 3.3กม10-3 g L-1
3.6. Linear range and detection limit
The peak current was proportional to the
concentration of resorcinol in the range of 5.5กม10-5~3.3กม10-3 g L-1
with correlation coefficient of 0.9986. The linear regression equation was Ip = 6.20กม10-3C
+ 0.12241, in which Ip and C indicated the peak current in mA and analyte
concentration in 10-5 g L-1, respectively. The detection limit of
the resorcinol in water was 1.1กม10-5 g L-1. The voltammetric
response of resorcinol was practically stable at least 1 h, with a maximum decrease less
than 5.0% being quite satisfactory for analytical purposes.
3.7. Interferences
The interferences from some metal ions, anions
and other organic compounds were examined with 5.5กม10-3 g L-1
resorcinol. Measurements of the peak current for each solution were repeated three times
and the average current values were obtained. If the presence of a compound altered the
average current of resorcinol less than กภ5%, then the compound was considered that did
not cause interference. The results showed that 100 folds of Mg2+, Ca2+,
K+, Na+, Mn2+, Cd2+, Cu2+, Br-,
NO3-, SO42-, CO32-, PO43-,
and 100 folds of phenol, methanol and ethanol had no obvious influence on the
determination of resorcinol. However, 2 folds excess of ascorbic acid, o-nitrophenol and
catechol caused the peak depression about 10%.
3.8. Practical analysis of samples
This method was applied to the determination of
resorcinol in samples of simulative wastewater and tap water respectively. The simulative
wastewater sample was constructed by adding resorcinol and co-existing components in tap
water, which contained 5.0กม10-4 g L-1 phenol, 5.0กม10-4 g
L-1 ethanol, 1.0กม10-4 mol L-1 NaCl, 1.0กม10-4 mol
L-1 Na2SO4 and different amount of resorcinol. The
concentration of resorcinol was determined by the standard addition method.
The determination of simulative wastewater and tap water was carried
respectively by CE method also to validate the proposed method. Results were listed in
Table 1. The recoveries and CE method suggested that the proposed method was effective for
the determination of resorcinol.
Table 1 Determination and results
recovery of samples
Sample |
Resorcinol in samples
(กม10-4g L-1) |
Added
(กม10-4g L-1) |
Found by proposed method
(กม10-4g L-1) |
Recovery
(%) |
Relative Standard Deviation (%) |
Found by CE method
(กม10-4g L-1) |
Tap water 1 |
0 |
5.0 |
4.76 |
95.2 |
4.74 |
4.80 |
Tap water2 |
0 |
10.0 |
10.48 |
104.8 |
5.09 |
10.12 |
Wastewater 1 |
3.8 |
5.0 |
8.68 |
98.6 |
3.18 |
8.60 |
Wastewater 2 |
4.2 |
10.0 |
14.47 |
101.9 |
4.39 |
14.21 |
4. CONCLUSIONS
The proposed method was an effective
method for the analysis of resorcinol. It was simple, accurate and precise, and time
saving. The determination of resorcinol on glassy carbon electrode modified by carbon
nanotube would be a choice method for resorcinol monitoring in wastewater.
REFERENCES
[1] Sangli A B, Kanabur V V, Environ. Ecol., 1998, 16 (3): 642-644.
[2] Cui H, Zhou J, Xu F, et al. Anal. Chim. Acta, 2004, 511 273-279.
[3] Wang L H, Kuo Y P. Chromatographia, 1999, 49: 208-211.
[4] Sharama O P, Bhat T K, Singh B. J. Chromatogr. A, 1998, 82 (1): 167-171.
[5] Khalaf K D, Hasan B A, Morales-Rubio A, et al. Talanta, 1994, 41 (4): 547-556.
[6] Onur F, Acar N. Int. J. Pharm., 1992, 78 (1-3): 89-91.
[7] Wright J D, Oliver J V. Sens. Actuators. B, 1998, 51 (1-3): 305-310.
[8] Bagirova N A, Shekhovtsova T N, van Huystee R B. Talanta, 2001, 55: 1151 -1164.
[9] Metzger J, Reiss M, Hartmeier W. Biosens. Bioelectron., 1998, 13 (10): 1077-1082.
[10] Kudryasheva N, Kratasyuk V, Esimbekova E, et al. Field. Anal. Chem. Technol., 1998, 2
(5): 277-280.
[11] Davis J J, Coles R J, Hill H A O. J. Electroanal. Chem., 1997, 440: 279-282.
[12] Katz E, Willner I, Wang J. Electroanal., 2004, 16: 19-44.
[13] Britto P J, Santhanam K S V, Ajayan P M. Bioelectrochem. Bioenerg., 1996, 41:
121-125.
[14] Ozkan S A, Ozkan Y, Senturk Z. J. Pharm. Biomed. Anal, 1998, 17: 299-305.
[15] Davis J J, Green M L H, Hill H A O, et al. Inorg. Chim. Acta, 1998, 272: 261-266.
[16] Luo H X, Shi Z J, Li N Q, et al. Anal. Chem., 2001, 73: 915-920.
[17] Nasr B, Abdellatif G, Canizares P, et al. Environ. Sci. Technol., 2005, 39:
7234-7239.
[18] Oliveira R T S, Salazar-Banda G R, Santos M C, et al. Chemosphere, 2007, 66:
2152-2158.
[19] Wang Z H, Li S J, Lv Q Z. Sensors and Actuators B, 2007, 127: 420-425.
กก
|